The Role of Thermodynamics in Power Generation: Key Concepts for Students!
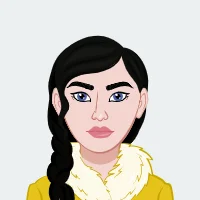
Thermodynamics stands as the bedrock of mechanical engineering, wielding profound significance in the understanding and application of energy-related processes. At its core, thermodynamics delves into the principles governing the conversion of energy from one form to another, a fundamental aspect that underpins the entire discipline. In the realm of mechanical engineering, where harnessing, converting, and optimizing energy holds paramount importance, a comprehensive grasp of thermodynamics is not just advantageous—it's indispensable. This is why tackling a thermodynamics assignment is crucial for students aiming to master these essential concepts and apply them effectively in their future careers.
The relevance of thermodynamics extends far beyond the theoretical confines of textbooks, finding tangible expression in the realm of power generation. Virtually every facet of power production, be it in conventional power plants, renewable energy systems, or cutting-edge technologies, is intricately entwined with thermodynamic principles. From the combustion of fossil fuels in steam power plants to the extraction of energy from renewable sources like wind and solar, the application of thermodynamics shapes the efficiency, sustainability, and overall performance of these power generation processes.
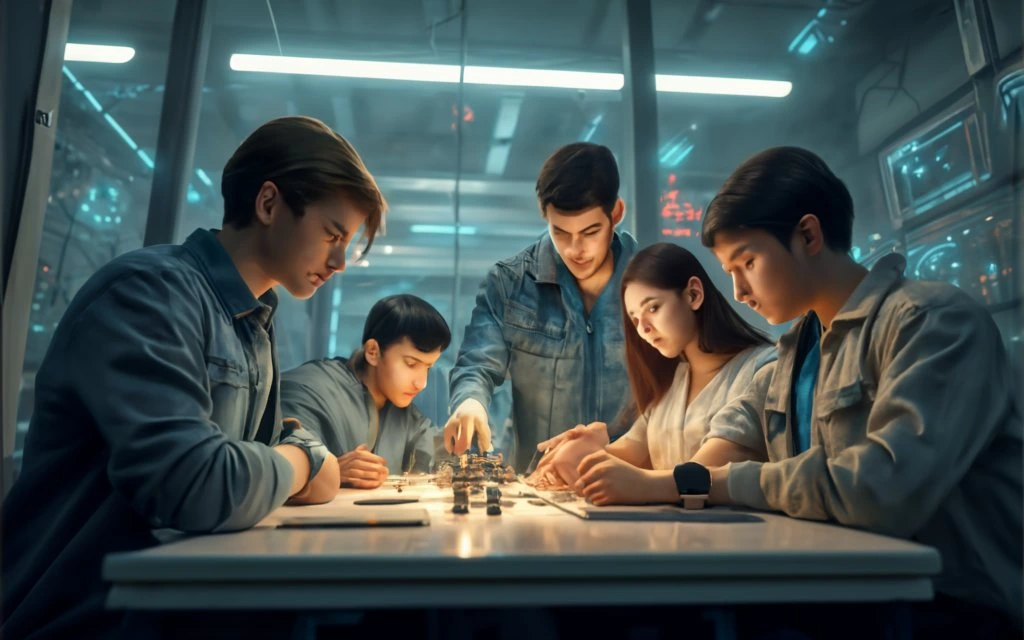
The impact of thermodynamics on various industries is profound and widespread. In power generation, thermodynamic principles dictate the design, efficiency, and environmental sustainability of energy-producing systems. In manufacturing, thermodynamics influences the efficiency of industrial processes, optimizing resource utilization and minimizing waste. In transportation, whether by land, sea, or air, the engines propelling vehicles adhere to the principles of thermodynamics, determining their fuel efficiency and overall performance.
One key concept that resonates prominently in the marriage of thermodynamics and power generation is the First Law of Thermodynamics, which asserts the conservation of energy. This principle underscores the crucial understanding that energy cannot be created nor destroyed; it can only be converted from one form to another. In the context of power generation, this law dictates the meticulous accounting of energy inputs and outputs, ensuring that the overall energy balance is maintained.
The Second Law of Thermodynamics introduces the concept of entropy, emphasizing the inherent tendency of systems to evolve towards disorder. In the realm of power generation, this law imposes limitations on the efficiency of energy conversion processes. It compels engineers and researchers to grapple with the challenges of increasing efficiency and minimizing energy losses, driving innovation in the design of power plants and renewable energy systems.
The application of these thermodynamic principles finds concrete expression in the Carnot, Rankine, and Brayton cycles. These theoretical frameworks provide the foundation for designing and optimizing power generation systems, whether they rely on steam in conventional power plants or gases in advanced gas turbine systems. Understanding these cycles is imperative for engineering students, as they serve as building blocks for comprehending the intricacies of real-world power generation processes.
In conclusion, the role of thermodynamics in power generation is not merely academic; it is a linchpin in the edifice of mechanical engineering. Its profound relevance to power generation and its far-reaching impact on diverse industries underscore the critical importance of students acquiring a robust understanding of thermodynamic principles. As they navigate the complexities of energy systems and contribute to the advancement of sustainable technologies, a solid foundation in thermodynamics becomes not just a theoretical asset but a practical necessity.
Basics of Thermodynamics
Thermodynamics, a cornerstone in the realm of physics and engineering, governs the principles that dictate the behavior of energy and its transformations within a system. At its core, thermodynamics deals with the concepts of energy, work, heat, and the fundamental laws that govern these processes.
Energy, the bedrock of thermodynamics, is the capacity to do work. In the context of thermodynamics, work refers to the application of force over a distance, resulting in the displacement of an object. This process involves the transfer or conversion of energy and is crucial in understanding how systems operate and transform energy.
Heat, another essential concept, is the transfer of thermal energy between two bodies at different temperatures. This transfer can occur through conduction, convection, or radiation, and it plays a pivotal role in various thermodynamic processes. Understanding heat is vital for analyzing how energy is exchanged within a system and how it influences its overall behavior.
The laws of thermodynamics form the guiding principles that govern energy interactions. The first law, often referred to as the law of conservation of energy, states that energy cannot be created or destroyed in an isolated system; it can only change forms. This foundational law emphasizes the importance of energy accounting and tracking its various forms as it moves through a system.
The second law delves into the quality of energy transformations. It introduces the concept of entropy, a measure of the system's disorder or randomness. This law asserts that, in any energy transformation, the total entropy of an isolated system tends to increase over time. It implies that some energy will be lost as unusable heat during the process, limiting the efficiency of energy conversions.
To comprehend these laws in practical scenarios, it's imperative to understand how thermodynamics is applied to various systems. Engineers and physicists often employ thermodynamic cycles as theoretical models to analyze energy transformations. One such cycle is the Carnot cycle, which sets an upper limit on the efficiency of heat-based systems. It serves as a benchmark for understanding the limitations of real-world thermodynamic processes.
The Rankine cycle, commonly employed in steam power plants, and the Brayton cycle, prevalent in gas turbine systems, provide practical insights into thermodynamics in power generation. These cycles showcase how energy is transformed and manipulated to generate power efficiently.
Thermodynamics in Power Generation
Thermodynamics plays a pivotal role in the realm of power generation, forming the cornerstone for the design and optimization of systems that convert heat into mechanical work. At its core, thermodynamics provides the theoretical framework to understand and harness energy transformations within these systems. The principles of thermodynamics guide engineers in creating efficient and sustainable power generation solutions that cater to the ever-growing global energy demand.
In the context of power generation, the first law of thermodynamics, the law of energy conservation, asserts that energy cannot be created nor destroyed; it can only change forms. This principle is foundational in designing power systems where the objective is to efficiently convert one form of energy, often heat, into useful mechanical work. Whether it's through traditional steam power plants or advanced gas turbine systems, engineers leverage thermodynamics to strike an optimal balance between energy input and output.
The second law of thermodynamics further refines the understanding of energy transformation. Entropy, a key concept derived from the second law, quantifies the degree of disorder in a system. Applying this principle to power generation, engineers seek to minimize entropy generation during energy conversion processes. By doing so, they enhance the efficiency of power plants and ensure that a significant portion of the input energy is effectively transformed into usable work.
The Carnot cycle, a theoretical construct representing the maximum efficiency attainable by a heat engine, serves as a benchmark for power generation systems. While no real-world system can achieve Carnot efficiency, it provides engineers with a target to approach through continuous improvement and innovation. The Rankine and Brayton cycles, commonly employed in steam and gas turbine power plants, respectively, are practical manifestations of thermodynamic principles in action. Engineers meticulously design and optimize these cycles to enhance efficiency, reliability, and environmental sustainability.
Real-world applications further underscore the integration of thermodynamics in power generation. From nuclear power plants to renewable energy sources like geothermal and solar, the principles of thermodynamics guide engineers in selecting and optimizing technologies for diverse energy landscapes. As the world transitions toward cleaner and more sustainable energy solutions, the role of thermodynamics becomes increasingly critical in designing systems that minimize environmental impact.
In essence, the marriage of thermodynamics and power generation is an intricate dance, where engineers navigate the complexities of energy conversion to meet the growing demands of society. The application of thermodynamic principles not only ensures the efficient utilization of resources but also paves the way for innovative solutions that redefine the future of power generation. As the quest for sustainable energy intensifies, the principles of thermodynamics remain steadfast as the guiding light in shaping the next generation of power systems.
Key Concepts for Students
In the realm of mechanical engineering, a profound comprehension of thermodynamics is imperative, particularly when delving into the intricate world of power generation. At its core, the First Law of Thermodynamics serves as the bedrock principle, embodying the conservation of energy. This fundamental concept posits that energy cannot be created nor destroyed but only transformed from one form to another. When applied to power generation processes, this law underscores the critical balance between the input and output energy, elucidating the necessity of optimizing efficiency to harness maximum power from available resources.
Conversely, the Second Law of Thermodynamics introduces the concept of entropy, a measure of energy dispersion in a system. In the context of power generation, entropy plays a pivotal role, dictating the directionality and efficiency of energy conversions. While the First Law emphasizes conservation, the Second Law introduces the stark reality of inherent limitations. Entropy tends to increase over time, leading to inefficiencies in power generation processes. Understanding these limitations is crucial for engineers striving to enhance the performance of systems and mitigate energy losses.
The Carnot Cycle emerges as a theoretical framework that embodies the pinnacle of efficiency in power generation. This cycle, although idealized, provides a benchmark for engineers to aspire to in designing systems that convert heat energy into mechanical work. By comprehending the intricacies of the Carnot Cycle, engineers can discern the theoretical limits of efficiency, guiding the practical applications of power generation systems toward optimal performance.
Moving from theory to practical application, the Rankine Cycle takes center stage in steam power plants. This cycle, widely employed in the industry, capitalizes on the transformation of water into steam to drive turbines and generate power. The Rankine Cycle, with its various stages and key components, exemplifies the practical integration of thermodynamic principles in large-scale power generation, underscoring the significance of these concepts in shaping the modern energy landscape.
Transitioning to gas turbine power plants, the Brayton Cycle showcases another facet of power generation efficiency. By introducing air compression, combustion, and expansion, the Brayton Cycle excels in applications where high power density and rapid response are paramount. Understanding the efficiency and practical considerations of the Brayton Cycle equips engineers with the knowledge to optimize gas turbine power plants, a crucial aspect in meeting the dynamic demands of contemporary energy needs.
In essence, these key thermodynamic concepts serve as guiding principles for mechanical engineering students navigating the intricate landscape of power generation. Mastering these principles not only unlocks a deeper understanding of the theoretical underpinnings but also empowers engineers to innovate and contribute to the sustainable and efficient generation of power in the real world.
Conclusion
In conclusion, this blog post has delved into the crucial role of thermodynamics in power generation, unraveling key concepts that form the backbone of this intricate field within mechanical engineering. At its essence, thermodynamics governs the transfer of energy, shaping the landscape of power generation processes across various industries. The fundamental principles, encapsulated by the laws of thermodynamics, provide a theoretical framework that engineers leverage to optimize efficiency and performance in power plants.
We've explored the cornerstone concepts that are paramount for any mechanical engineering student aiming to comprehend the intricate dance of energy within power generation systems. The First Law of Thermodynamics, anchoring the principle of energy conservation, lays the groundwork for understanding the fundamental nature of energy transformations. Meanwhile, the Second Law, introducing the concept of entropy, adds layers of complexity, outlining the limitations and possibilities inherent in these dynamic processes.
Delving further, we've examined the theoretical underpinnings of power cycles – the Carnot, Rankine, and Brayton cycles – each serving as a blueprint for the design and operation of diverse power generation systems. From steam power plants to gas turbine facilities, these cycles are the practical manifestations of thermodynamics principles, illustrating the tangible impact of theoretical knowledge on real-world applications.
In acknowledging the importance of these concepts for mechanical engineering students, it becomes evident that a solid grasp of thermodynamics is indispensable. Not only does it lay the groundwork for academic success, but it also serves as a compass for navigating the challenges posed by the ever-evolving landscape of power generation technologies.
As readers, you are encouraged to delve deeper into these concepts, exploring real-world examples and case studies that exemplify the symbiotic relationship between thermodynamics and power generation. Further resources, academic materials, and in-depth studies can provide a more comprehensive understanding, nurturing your curiosity and intellectual growth in this field.
Should the intricacies of thermodynamics pose challenges or if you seek additional assistance in exploring these concepts further, our dedicated services at mechanicalengineeringassignmenthelp.com are readily available. Our team of experts stands ready to assist you on your academic journey, providing invaluable insights and guidance to ensure your success in grasping the nuances of thermodynamics and its applications in power generation. Your academic excellence is our priority, and we invite you to reach out, explore, and thrive in the fascinating world of thermodynamics within mechanical engineering.