The Unsung Heroes of Mechanical Engineering: Lesser-Known Principles Every Student Should Know before Solving Assignments
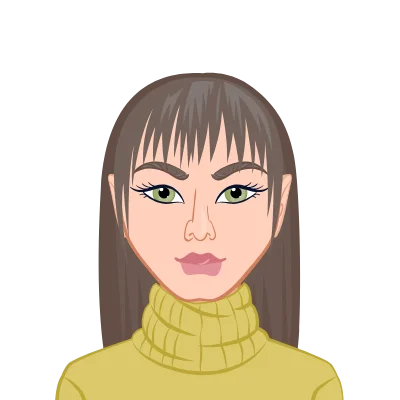
Mechanical engineering is a vast field, an expansive landscape echoing with the resonance of principles that serve as the unsung heroes, intricately woven into the fabric of countless innovations. Beyond the well-trodden paths of familiar concepts, there exists a trove of lesser-known principles, quietly orchestrating the symphony of mechanical marvels. As a student navigating the terrain of assignments, these principles emerge as hidden keys, unlocking doors to new perspectives and offering solutions to the complexity that defines mechanical engineering challenges. This blog embarks on a journey to illuminate these concealed gems, shedding light on their significance and unveiling their relevance in the grand tapestry of mechanical engineering. Each principle, though less heralded, plays a pivotal role, contributing to the very essence of innovation that defines the field. Prepare to delve into the depths of these concealed truths, where the seemingly obscure principles become beacons guiding students and enthusiasts alike through the unexplored realms of mechanical engineering, enriching their understanding and broadening their horizons. If you seek assistance with your Mechanical Engineering assignment, these principles can serve as invaluable tools in comprehending the intricate puzzles awaiting exploration.
Hysteresis: Unraveling the Hidden Loops
In the intricate tapestry of mechanical engineering, the phenomenon of hysteresis stands as an often-overlooked thread. Delving into the unseen complexities of this principle reveals a world where the output of a system trails behind its input, creating elusive loops in the response. This concept, paramount in materials science, holds particular significance for engineers navigating the design of springs, dampers, and other mechanical components. As students embark on the journey of assignments, understanding hysteresis becomes a key to deciphering the behavior of materials under varying loads. Unravel the hidden loops of hysteresis, and you'll find yourself equipped with insights essential for crafting mechanical systems that dance in harmony with the intricate rhythms of their environments.
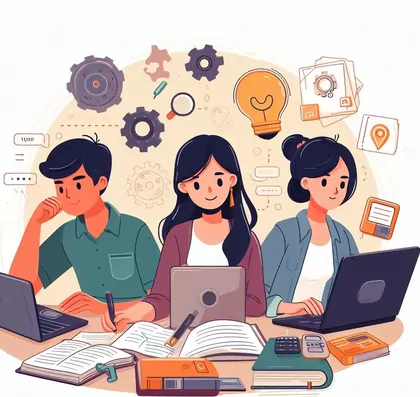
Understanding Hysteresis
Unveiling its significance in the intricate landscape of mechanical engineering, hysteresis emerges as a lesser-known yet pivotal principle deserving of attention. This phenomenon, where a system's output lags behind its input, paints a picture of intricate loops in the response—a subtlety often overlooked. In the realm of materials science, hysteresis exercises its influence, shaping the behavior of materials under the ebb and flow of varying loads. Particularly manifest in mechanical components such as springs and dampers, students navigating the terrain of assignments encounter hysteresis as a silent orchestrator. Grasping its effects becomes paramount, akin to deciphering a hidden language, for it holds the key to designing mechanical systems that perform with optimal precision and efficiency, dancing in harmony with the demands of their environments.
Practical Applications of Hysteresis
Navigating the labyrinth of mechanical engineering applications, hysteresis emerges as a guiding principle with multifaceted implications.
- Material Selection: In the intricate dance of material selection, engineers find hysteresis to be a crucial partner, especially in applications prone to cyclic loading. Beyond the surface-level considerations, delving into hysteresis allows for the identification of materials that exhibit optimal behavior under repetitive stresses. This deeper understanding aids in the selection of materials for machinery components, ensuring durability and performance over extended operational cycles.
- Vibration Damping: The realm of vibration damping unfolds as a canvas where hysteresis paints an essential stroke. Beyond its theoretical implications, hysteresis becomes a practical tool for engineers sculpting effective vibration dampers. By leveraging the controlled dissipation of energy inherent in hysteresis, engineers create damping systems that ensure vibrations are quelled in a precisely regulated manner. This not only safeguards machinery from excessive oscillations but also contributes to the overall efficiency and longevity of mechanical systems.
Gait Analysis: Walking into Biomechanics
Venturing beyond the traditional confines of mechanical engineering, the realm of gait analysis emerges as a captivating exploration into biomechanics. This unsung hero principle involves the meticulous study of human walking patterns, unraveling the intricate dance of muscles, joints, and forces. In the synthesis of biomechanics and mechanical engineering, gait analysis serves as a beacon, guiding engineers in the creation of prosthetics and robotics that seamlessly integrate with the natural cadence of human movement. As students delve into the nuances of assignments, understanding the principles of gait analysis becomes a gateway to designing assistive technologies that not only mimic but enhance the grace and efficiency of human locomotion. Step into the world of gait analysis, where every stride unveils the secrets that bridge the gap between mechanics and the poetry of motion.
The Science Behind Gait Analysis
Venturing into the crossroads of biomechanics and traditional mechanical engineering, the exploration of gait analysis unveils a realm where seemingly distant principles converge with profound implications. Though biomechanics may appear a departure from the conventional, its principles form an integral part of cutting-edge fields such as prosthetics and robotics. Gait analysis, a beacon in this convergence, delves into the meticulous study of human walking patterns. Engineers, armed with insights from gait analysis, embark on a transformative journey, using this data to craft devices that not only replicate but elevate natural movements. As students navigate assignments, understanding the intricacies of gait becomes a compass guiding them towards the synthesis of mechanics and biology—a space where assistive technologies seamlessly integrate with the complex poetry of the human body, creating innovations that transcend the boundaries of traditional engineering disciplines.
Key Parameters in Gait Analysis
Embarking on the journey into the intricacies of gait analysis, engineers unearth key parameters that serve as the building blocks for transformative innovations.
- Stride Length and Frequency: Delving beyond the surface of human locomotion, engineers immerse themselves in the significance of stride length and frequency. These parameters transcend mere metrics, becoming the foundation for the design of prosthetics and exoskeletons. By understanding and optimizing these aspects, engineers craft assistive technologies that not only restore natural walking but enhance efficiency, offering individuals a seamless integration of technology with their innate movements.
- Joint Angles: The symphony of human walking is composed of subtle joint movements, each contributing to the fluidity of motion. In the realm of gait analysis, engineers embrace the role of joint angles as pivotal elements. Through meticulous analysis, the data on joint angles becomes a blueprint for the development of robotic systems. These systems, finely tuned to replicate human movements, transcend mere imitation, offering a glimpse into a future where robotics seamlessly mirrors the grace and precision of human ambulation.
Pareto Principle in Design Optimization: The 80/20 Rule
In the orchestration of design optimization, the Pareto Principle emerges as a silent maestro, conducting the harmonious balance between effort and impact. Recognized as the 80/20 rule, this unsung hero principle asserts that a crucial 20% of factors contribute to 80% of the outcomes. As students navigate the complexities of mechanical engineering assignments, embracing the Pareto Principle becomes a strategic compass. By identifying and prioritizing the vital few factors, engineers streamline the design process, channeling efforts where they yield maximal impact. This principle transcends numerical ratios; it becomes a guiding philosophy, enabling efficient decision-making and resource allocation. Step into the realm of design optimization guided by the Pareto Principle, where the pursuit of excellence is not about doing more but doing the right things—the 20% that catalyze 80% of success.
Unveiling the Power of the Pareto Principle
In the intricate dance of design optimization within mechanical engineering, the Pareto Principle emerges as a potent orchestrator, wielding the philosophy encapsulated in the famed 80/20 rule. A concept named after economist Vilfredo Pareto, this principle succinctly proposes that a staggering 80% of effects can be traced back to a mere 20% of causes. Within the realm of mechanical engineering assignments, the application of the Pareto Principle becomes a strategic compass. Engineers, akin to artisans sculpting a masterpiece, can streamline the design process by identifying and honing in on the critical factors that wield the most substantial influence. As students embark on assignments, understanding the power encapsulated within this principle becomes a transformative lens, enabling a focused approach where efficiency is not about doing more but about channeling efforts precisely where they yield the most significant impact—a key to unlocking the door to design excellence.
Applying the Pareto Principle in Mechanical Engineering
In the intricate tapestry of mechanical engineering, the Pareto Principle becomes a guiding philosophy, directing engineers towards precision and efficiency in crucial domains.
- Material Selection: Beyond the labyrinth of material options, the application of the Pareto Principle unveils a streamlined approach. Engineers sift through the plethora of materials, identifying the vital 20% that embodies 80% of the required mechanical properties. This focused lens transforms material selection from a daunting task to a strategic endeavor, simplifying decision-making and ensuring that the chosen materials align with optimal performance criteria.
- Fault Diagnosis: When the heartbeat of mechanical systems falters, the Pareto Principle serves as a diagnostic compass. Rather than drowning in a sea of potential issues, engineers pinpoint the critical 20% of problems responsible for 80% of the system's troubles. This targeted approach to fault diagnosis streamlines troubleshooting processes, accelerating the identification and resolution of issues, thereby optimizing system reliability and minimizing downtime.
Chaotic Vibrations: Embracing the Unpredictable
Within the symphony of mechanical vibrations, an unconventional melody emerges with chaotic vibrations at its core. This unsung hero principle challenges the conventional notion of predictability, inviting engineers to embrace the beauty of controlled chaos. In the dance of unpredictability, chaotic vibrations present opportunities rather than obstacles. Students exploring this principle in mechanical assignments uncover a realm where randomness can be harnessed for practical applications. From converting chaotic vibrations into usable energy to employing controlled chaos for random number generation, this principle reshapes the narrative of mechanical engineering. It transforms the unpredictable into a tool, opening doors to innovation and efficiency. Embrace the enigma of chaotic vibrations, and witness how the seemingly disorderly can be a powerful force, steering mechanical systems towards new frontiers of functionality and adaptability.
Exploring Chaos in Mechanical Vibrations
Within the rhythmic realm of mechanical vibrations, chaos unfolds as an unconventional yet potent force, challenging preconceived notions of negativity. Far from being a disruptive entity, chaotic vibrations, marked by their unpredictable nature, unveil a realm of possibilities for engineers. In the nuanced understanding of chaotic behavior within mechanical systems, a transformative narrative emerges. Engineers wielding this understanding embark on a journey to design devices that don't merely navigate chaos but harness it for enhanced performance. Chaos, when controlled, becomes a tool for innovation, a force that propels mechanical systems into realms of efficiency and adaptability previously unexplored. As students delve into assignments, the exploration of chaotic vibrations becomes a gateway to a paradigm where unpredictability is not a hindrance but a strategic advantage—an unsung hero in the orchestration of mechanical systems toward new frontiers of functionality and resilience.
Harnessing Chaos for Practical Applications
In the seemingly turbulent landscape of chaotic vibrations, engineers discover a realm where disorder becomes a wellspring of opportunity, presenting avenues for innovative applications.
- Energy Harvesting: Beneath the surface of chaos lies a latent potential waiting to be harnessed. Engineers exploring chaotic vibrations unveil a fascinating prospect—energy harvesting. By converting chaotic vibrations into usable energy, this principle opens doors to the development of self-powered sensors and devices. In the midst of apparent randomness, a structured means of energy generation emerges, offering sustainable solutions that transcend traditional power sources.
- Random Number Generation: Chaos, when harnessed and controlled, transforms into a tool essential in the realm of random number generation. In the intricate dance of engineering simulations and algorithms, the controlled chaos within chaotic systems becomes a reliable source for generating truly random numbers. This application extends beyond mere unpredictability; it becomes a cornerstone in the foundation of simulations, ensuring accuracy and authenticity in diverse engineering scenarios where randomness is a critical element.
Conclusion
In the expansive realm of mechanical engineering, the culmination of these lesser-known principles serves as a testament to their status as unsung heroes, silently but significantly shaping the trajectory of design decisions and paving the way for future innovation. As a student poised to confront the challenges of assignments, immersing yourself in the embrace of these concepts is akin to donning a unique lens that unveils hidden dimensions within mechanical systems. This immersion not only fosters a profound understanding but also positions you as a discerning engineer with a distinctive perspective. Beyond the theoretical realms of hysteresis, gait analysis, the Pareto Principle, and chaotic vibrations lie practical applications that beckon exploration. It's an invitation to not only comprehend these principles but to actively engage with them, applying their nuances to real-world scenarios. So, embark on the journey into the intricate world of mechanical engineering, where these unsung heroes are not just guides but companions propelling you to unparalleled heights in your academic and professional journey, where innovation and understanding converge to shape the future of the field.