Advanced Studies in Fluid Thermodynamics: Critical Point Analysis and Applications
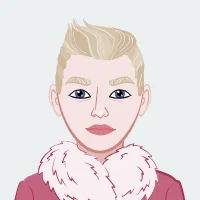
Fluid thermodynamics is a cornerstone of mechanical engineering, serving as a fundamental discipline that underpins the design, analysis, and optimization of a myriad of systems. At its core, fluid thermodynamics explores the behavior of fluids—liquids and gases—under different temperature, pressure, and volume conditions. In the realm of mechanical engineering, this knowledge is indispensable for comprehending the intricacies of systems ranging from power plants to aerospace propulsion. If you need help with your Thermodynamics assignment, understanding fluid thermodynamics is crucial for mastering the principles and applications of thermodynamics in mechanical engineering.
Critical to the study of fluid thermodynamics is the concept of critical points, which represent unique states at which a substance undergoes distinct changes in its physical properties. These critical points are characterized by specific combinations of temperature and pressure, beyond which the substance transitions between liquid and gas phases becomes seamless. Understanding these critical points is paramount due to their far-reaching implications across various engineering applications.
In the context of power generation, comprehending critical points is essential for optimizing steam power cycles. At the critical point, the distinction between liquid and vapor phases diminishes, leading to significant changes in thermodynamic properties. Engineers leverage this understanding to design more efficient power plants, enhancing energy conversion processes and minimizing waste. Similarly, in refrigeration systems, knowledge of critical points aids in the development of cooling systems that operate with increased efficiency and reduced environmental impact.
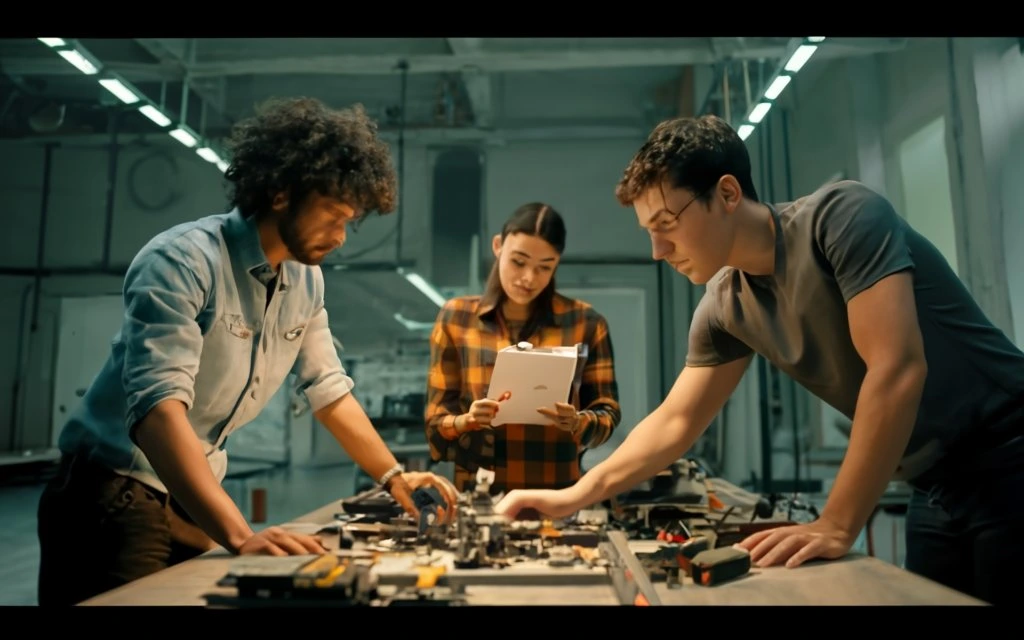
Aerospace engineering is another domain where fluid thermodynamics and critical point analysis converge to drive innovation. Propulsion systems, such as those in rockets and jet engines, rely on precise control of fluid dynamics. At critical points, fluids exhibit unique behaviors that impact thrust and efficiency. Engineers exploit this knowledge to optimize combustion processes and enhance the overall performance of aerospace vehicles, ensuring they operate reliably in diverse conditions.
Furthermore, in industrial processes, the applications of critical point analysis are widespread. From chemical manufacturing to material processing, engineers must grapple with the complexities of fluid behavior. By understanding critical points, they can manipulate conditions to achieve desired outcomes, whether it be in the synthesis of chemicals or the fabrication of materials with specific properties.
The importance of critical point analysis in fluid thermodynamics extends beyond the immediate applications. It serves as a cornerstone for the development of experimental techniques and methodologies. Researchers and engineers employ specialized equipment and innovative approaches to precisely determine critical points in different substances. This empirical knowledge, coupled with theoretical understanding, contributes to the ongoing refinement of fluid thermodynamics models and enhances the predictive capabilities of engineers.
In conclusion, fluid thermodynamics, with a focus on critical point analysis, holds a pivotal role in mechanical engineering. The applications are vast and varied, influencing the design and optimization of systems that power our cities, propel our vehicles, and shape our industrial landscape. As we delve deeper into the complexities of fluid behavior, the understanding of critical points emerges as a linchpin, enabling engineers to navigate the challenges of an ever-evolving technological landscape. The pursuit of knowledge in this realm not only unlocks new possibilities but also ensures that mechanical engineering continues to push the boundaries of innovation.
Definition of Critical Point:
In fluid thermodynamics, a critical point is a defining state at which a substance undergoes distinctive changes in its physical properties. This critical point represents the culmination of the phase diagram, specifically where the boundary between liquid and gas phases ceases to exist, resulting in a singular phase known as the critical phase. At this juncture, both the liquid and gas states exhibit identical properties, such as density and refractive index, marking a point of unique equilibrium.
The conditions leading to the formation of a critical point are contingent upon the interplay of temperature and pressure. Typically, as a substance experiences an increase in temperature and pressure, its liquid and gas phases become less distinguishable. However, it is at the critical point that these phases converge, and the substance manifests properties characteristic of both phases simultaneously. The critical temperature represents the temperature at which this convergence occurs, while the critical pressure denotes the pressure needed to maintain this equilibrium.
As a substance approaches its critical point, there is a noticeable departure from the familiar behavior observed in the liquid and gas phases. Near the critical point, substances display peculiarities, such as enhanced compressibility and the absence of a distinct meniscus between liquid and gas. The critical point also heralds significant changes in thermodynamic properties. For instance, the critical density, which is the density at the critical point, becomes uniform for both liquid and gas. Moreover, the critical pressure and temperature define the boundaries beyond which the substance cannot exist in distinct liquid or gas states, making the critical point a transformative threshold in fluid thermodynamics.
The critical point's significance extends beyond theoretical considerations, finding practical applications in various engineering disciplines. Engineers leverage this understanding to optimize processes in power plants, design more efficient refrigeration systems, and enhance the performance of propulsion systems in aerospace applications. Moreover, researchers employ experimental techniques to precisely determine the critical point, contributing to the development of accurate thermodynamic models and fostering advancements in fluid dynamics. In essence, the critical point is a pivotal concept in fluid thermodynamics, marking a juncture where substances undergo a unique transition, and its comprehension is paramount for engineers and scientists seeking to unlock the full potential of fluid systems in diverse applications.
Importance of Critical Point Analysis:
Studying critical points in fluid thermodynamics holds profound significance as it serves as a linchpin for the design and optimization of various mechanical systems crucial to our technological infrastructure. The analysis of critical points offers engineers and researchers valuable insights into the behavior of fluids under extreme conditions, enabling them to enhance the efficiency, reliability, and sustainability of mechanical systems.
In the realm of power generation, understanding critical points is paramount for optimizing steam power cycles. At the critical point, the properties of water undergo significant changes, impacting the efficiency of energy conversion processes. Engineers leverage this knowledge to fine-tune the design of power plants, ensuring that they operate at maximum efficiency. This optimization not only improves the overall performance of power generation systems but also contributes to the reduction of environmental impact by minimizing energy losses.
Heat exchangers, integral components in various industrial processes, benefit immensely from the analysis of critical points. These devices facilitate the transfer of heat between fluids at different temperatures. By considering critical points, engineers can design heat exchangers with improved heat transfer characteristics, leading to more efficient energy utilization and reduced operational costs. This optimization is particularly relevant in industries where precise temperature control is essential, such as chemical manufacturing and food processing.
In the field of propulsion systems, whether for aircraft, rockets, or other vehicles, critical point analysis plays a crucial role. The behavior of fluids at and around critical points influences combustion processes and thrust generation. Engineers leverage this knowledge to design propulsion systems that operate with greater efficiency and reliability. By understanding the unique characteristics of fluids near critical points, they can fine-tune the combustion parameters, resulting in enhanced performance and fuel efficiency.
Furthermore, the application of critical point analysis extends to environmental considerations. By optimizing mechanical systems based on insights derived from critical points, engineers contribute to the development of sustainable technologies. This is particularly relevant in the current era where environmental concerns drive the imperative for cleaner and more efficient energy solutions.
In essence, the significance of studying critical points lies in its transformative impact on the design and optimization of mechanical systems. From power plants to heat exchangers and propulsion systems, the insights gained through critical point analysis empower engineers to create more efficient, reliable, and environmentally friendly technologies. As we continue to push the boundaries of innovation in mechanical engineering, the understanding of critical points stands as a cornerstone for shaping a sustainable and technologically advanced future.
Applications in Mechanical Engineering:
Critical point analysis in fluid thermodynamics finds diverse and specific applications in various facets of mechanical engineering, significantly impacting the design and operation of critical systems. In steam power cycles, a fundamental application of critical point analysis lies in optimizing the performance of steam turbines. At the critical point, water and steam properties undergo drastic changes, affecting the efficiency of the energy conversion process. Engineers meticulously consider critical conditions to design power plants that operate at maximum efficiency, leading to enhanced power generation and reduced environmental impact.
Refrigeration systems, pivotal in maintaining temperature-controlled environments in industries and households, benefit greatly from critical point analysis. By understanding the critical points of refrigerants, engineers can tailor the design of these systems for optimal efficiency. The behavior of refrigerants near their critical points influences heat transfer characteristics, enabling the creation of more efficient and environmentally friendly refrigeration technologies. This application ensures precise temperature control in processes ranging from food preservation to industrial manufacturing.
In industrial processes, critical point analysis proves invaluable for manipulating fluid behavior to achieve desired outcomes. For instance, in chemical manufacturing, where precise control over reaction conditions is essential, understanding critical points aids in optimizing reaction kinetics and product yields. Engineers leverage this knowledge to design systems that operate under conditions near critical points, enhancing the efficiency and reliability of chemical processes.
The behavior of fluids near critical points also plays a pivotal role in supercritical fluid technology, an emerging field with applications in industrial extraction and materials processing. Supercritical fluids, operating near their critical points, exhibit unique properties that make them highly effective solvents. Engineers utilize critical point analysis to optimize extraction processes, facilitating the separation of desired compounds from raw materials in a more environmentally friendly and efficient manner.
Moreover, in the field of material science and manufacturing, critical point analysis contributes to processes like supercritical fluid drying and impregnation. These applications harness the unique properties of fluids near their critical points to achieve precise control over the fabrication and modification of materials, ensuring desired characteristics such as porosity and strength.
In summary, critical point analysis in mechanical engineering extends its influence across diverse applications, from steam power cycles and refrigeration systems to industrial processes and material science. The ability to harness the transformative behavior of fluids near critical points empowers engineers to design more efficient, reliable, and environmentally conscious technologies across a spectrum of mechanical engineering disciplines.
Experimental Techniques:
Determining critical points in fluid thermodynamics requires a combination of sophisticated experimental techniques, meticulous data analysis, and the utilization of specialized equipment. Laboratory experiments play a pivotal role in this process, often involving the controlled manipulation of temperature and pressure conditions to observe the behavior of a substance as it approaches its critical point. One common experimental approach is the isochoric method, where the volume of the fluid remains constant, and researchers carefully measure the changes in temperature and pressure. Another method involves the isobaric technique, maintaining constant pressure while varying temperature. These experiments provide crucial data points, allowing scientists to construct phase diagrams and identify the critical region.
Specialized equipment is essential for conducting precise experiments related to critical point determination. High-pressure vessels and reactors capable of withstanding extreme conditions are employed to create environments where substances reach their critical points. Piston-cylinder apparatus and variable-volume view cells are examples of tools used to maintain specific conditions for critical point analysis. Additionally, specialized sensors and detectors, such as those measuring refractive index or acoustic properties, are employed to capture subtle changes in the substance's behavior as it approaches critical conditions.
Data analysis is a critical aspect of experimental determination, involving the interpretation of collected data to pinpoint the exact values of critical temperature and pressure. Researchers employ mathematical models and statistical methods to analyze the experimental results, identifying trends and correlations indicative of critical behavior. Curve fitting techniques, such as the Van der Waals equation or the Redlich-Kwong equation, are often applied to experimental data to extract precise critical point values.
Modern advancements in technology have facilitated more sophisticated experimental approaches, including spectroscopic techniques. Infrared spectroscopy, Raman spectroscopy, and neutron scattering methods provide detailed insights into molecular interactions as substances approach their critical points. These techniques allow researchers to probe the subtle changes in molecular structure and dynamics, contributing to a deeper understanding of the critical phenomena.
Moreover, computer simulations, utilizing molecular dynamics or Monte Carlo methods, complement experimental approaches by providing additional insights into the behavior of fluids near critical points. These simulations enable researchers to explore a wide range of conditions and study critical phenomena in virtual environments.
In conclusion, the determination of critical points involves a combination of laboratory experiments, specialized equipment, and advanced data analysis techniques. The synergy of these elements enables researchers to unlock the mysteries of fluid behavior near critical points, paving the way for advancements in fluid thermodynamics and the broader field of mechanical engineering.
Case Studies:
Several real-world case studies highlight the transformative impact of a deep understanding of critical points in fluid thermodynamics, leading to significant advancements and improvements in various mechanical systems. One notable example is in the field of supercritical fluid technology, where a profound grasp of critical points has revolutionized industrial processes.
In pharmaceutical manufacturing, the application of supercritical fluid extraction (SFE) has emerged as a game-changer. Traditional extraction methods often involve the use of organic solvents, posing environmental and safety concerns. Supercritical fluids, operating near their critical points, offer an alternative. Carbon dioxide, commonly used in SFE, exhibits both liquid and gas-like properties near its critical point, allowing it to penetrate materials like a gas while dissolving solutes like a liquid. This has led to more efficient and environmentally friendly extraction processes for pharmaceutical compounds, ensuring high purity and minimizing environmental impact.
Another compelling case study lies in the realm of materials processing. The understanding of critical points has facilitated advancements in the production of aerogels, highly porous and lightweight materials with diverse applications. Supercritical drying, a process that exploits the unique properties of fluids near critical points, allows for the creation of aerogels with controlled structures and properties. This method ensures the removal of the liquid component from the gel without causing structural collapse, resulting in aerogels with enhanced thermal insulation properties used in applications ranging from aerospace to oil and gas.
In the energy sector, particularly in the optimization of power plants, critical point analysis has led to significant improvements. The design of advanced steam cycles, leveraging insights into critical points, has increased the efficiency of power generation. Supercritical and ultra-supercritical steam conditions, operating near or beyond the critical point of water, allow for higher efficiency and lower greenhouse gas emissions. This has a direct impact on the sustainability and environmental footprint of power generation, addressing the global demand for cleaner and more efficient energy solutions.
Furthermore, advancements in propulsion systems, such as those in the aerospace industry, owe much to the understanding of critical points. The optimization of combustion processes in jet engines relies on precise control over fluid dynamics near critical conditions, leading to increased thrust and fuel efficiency. This has not only enhanced the performance of aircraft but has also contributed to the development of more sustainable and environmentally friendly aviation technologies.
In conclusion, the real-world applications of a deep understanding of critical points in fluid thermodynamics are diverse and impactful. From pharmaceutical manufacturing to materials processing, and from power generation to aerospace propulsion, advancements driven by critical point analysis have ushered in a new era of efficiency, sustainability, and innovation in mechanical systems.
Conclusion:
The blog post delves into the intricacies of fluid thermodynamics, particularly focusing on the critical points of substances, and highlights its pivotal role in various mechanical engineering applications. The significance of understanding critical points is underscored in the context of power generation, with optimized steam power cycles enhancing the efficiency of power plants and reducing environmental impact. In refrigeration systems, critical point analysis contributes to the design of more efficient cooling technologies, ensuring precise temperature control in diverse applications. The aerospace industry benefits from insights into critical points as well, where the optimization of propulsion systems relies on a deep understanding of fluid dynamics near critical conditions, resulting in increased efficiency and sustainability in aviation.
The exploration extends to industrial processes, where critical point analysis proves instrumental in chemical manufacturing. Engineers leverage this understanding to optimize reaction conditions, enhancing the efficiency and reliability of chemical processes. The emerging field of supercritical fluid technology showcases the transformative impact of critical point analysis in applications such as pharmaceutical extraction and materials processing. These advancements highlight the versatility of critical point knowledge in addressing real-world challenges across various domains.
The blog also sheds light on the experimental techniques and specialized equipment employed to determine critical points. Laboratory experiments, using methods like isochoric and isobaric techniques, alongside specialized equipment such as high-pressure vessels and reactors, are crucial in creating conditions where substances reach their critical points. Data analysis techniques, including curve fitting and mathematical models, complement these experiments to extract precise critical point values. Modern advancements in technology, such as spectroscopic techniques and computer simulations, further enhance the depth and accuracy of critical point analysis.
The case studies presented exemplify how a profound understanding of critical points leads to groundbreaking advancements. From the development of cleaner pharmaceutical extraction methods to the creation of advanced materials like aerogels, and the optimization of power plants and propulsion systems, the impact on technology and sustainability is evident. The emphasis on ongoing research and advancements in fluid thermodynamics is a recurring theme throughout the blog. It underscores the dynamic nature of the field and the constant quest for deeper insights and innovative applications. The evolving landscape of mechanical engineering relies on the continuous exploration of critical points, driving the development of more efficient, sustainable, and technologically advanced systems. In essence, the blog advocates for a sustained commitment to fluid thermodynamics research, recognizing it as a cornerstone for shaping the future of mechanical engineering.