The Essentials of Control Systems Engineering: Assignment Guidance for Aspiring Engineers
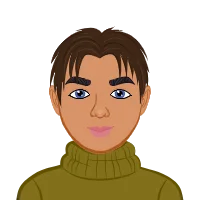
In the dynamic realm of mechanical engineering, where innovation and precision intersect, the discipline of control systems engineering stands as a cornerstone. Aspiring engineers delve into the intricate world of control systems to master the art of managing and manipulating dynamic systems. This blog, titled "The Essentials of Control Systems Engineering: Assignment Guidance for Aspiring Engineers," seeks to unravel the complexities of this field and provide invaluable insights to students navigating the challenges of control systems assignments. Whether you are seeking help with your control systems assignment or aiming to grasp the fundamental concepts of this discipline, this blog serves as a comprehensive guide to support your journey in mastering the intricacies of control systems engineering.
Control systems engineering, a vital subset of mechanical engineering, is a multifaceted discipline that orchestrates the behavior of dynamic systems to achieve desired outputs. This blog aims to be a guiding beacon for students, offering a comprehensive exploration of key concepts, common challenges, and practical tips essential for conquering control systems assignments. By addressing the intricate nuances of mathematical modeling, controller design, and system response analysis, this blog endeavors to demystify the subject matter, empowering aspiring engineers to approach their assignments with confidence.
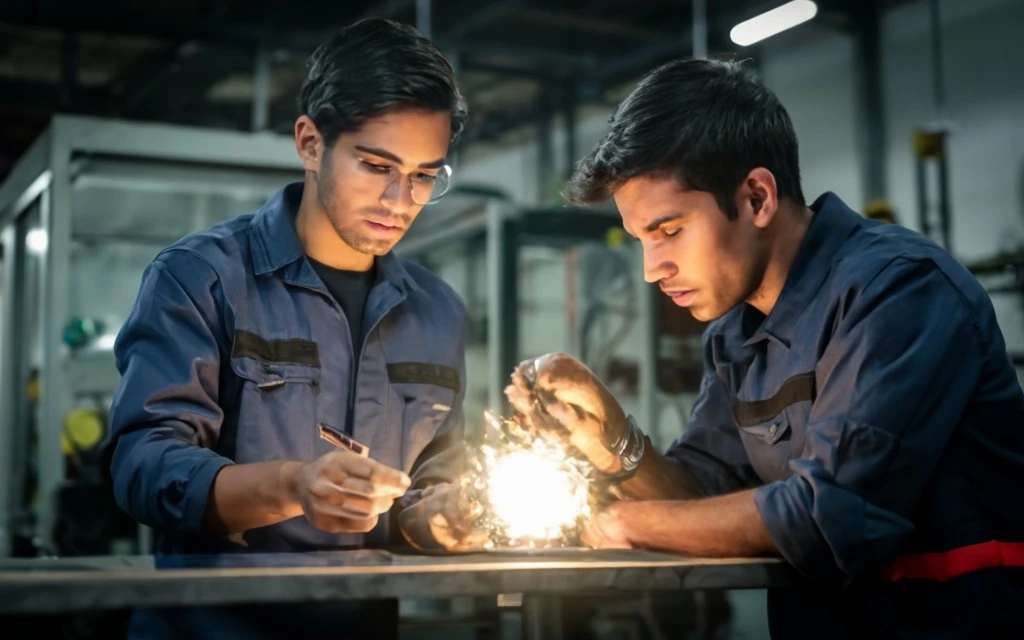
In the initial section, we embark on a journey to understand the fundamental principles of control systems engineering. From defining the scope of this discipline to elucidating key concepts like feedback, stability, and system dynamics, the blog lays a solid foundation for readers to grasp the essence of control systems. Real-world examples seamlessly weave through the narrative, providing a tangible connection between theoretical concepts and practical applications.
The subsequent section delves into the common challenges that students often encounter when tackling control systems assignments. Mathematical modeling, a crucial aspect of control systems engineering, can be a daunting task for many. This blog provides insightful tips and tricks, guiding students through the process of effectively modeling physical systems. Controller design, another intricate facet, is demystified with discussions on selecting appropriate control strategies. The analysis of system response, often a stumbling block for learners, is approached with clarity, accompanied by step-by-step solutions and illustrative examples.
Recognizing the importance of practicality in engineering education, the third section offers tips for success in control systems assignments. From advocating for the effective use of software tools to exploring real-world applications and providing case studies, the blog bridges the gap between theory and application. It not only equips students with the necessary theoretical knowledge but also instills a practical understanding of how control systems engineering manifests in industries.
In conclusion, this blog serves as a comprehensive guide for aspiring engineers navigating the intricate landscape of control systems engineering assignments. By providing a holistic perspective on key concepts, addressing common challenges, and offering practical tips, it aspires to be a go-to resource for students seeking clarity and mastery in this vital discipline. As readers embark on this exploration, they are encouraged to delve further into the realm of mechanical engineering assignment help offered at mechanicalengineeringassignmenthelp.com, where expertise meets academic success.
Understanding Control Systems Engineering.
Control Systems Engineering, at its core, represents the mastery of orchestrating and regulating the behavior of dynamic systems to achieve specific objectives. In the vast domain of mechanical engineering, understanding control systems is akin to navigating the intricate dance of variables, inputs, and outputs within a myriad of applications. Broadly defined, control systems involve the manipulation of systems to ensure they respond predictably to desired inputs, encompassing a diverse range of industries such as aerospace, automotive, and manufacturing. This blog endeavors to unravel the layers of complexity surrounding control systems engineering, providing aspiring engineers with a profound comprehension of its fundamental principles.
At the heart of control systems lies the concept of feedback, a critical element that distinguishes these systems from their open-loop counterparts. Feedback mechanisms enable the system to continually adjust its behavior based on the output, maintaining stability and accuracy. Stability, another key concept, delves into the system's ability to return to a balanced state after encountering disturbances. System dynamics, the study of how systems evolve over time, completes the triad of fundamental principles. These foundational concepts set the stage for a deeper exploration into the intricate workings of control systems.
Real-world applications serve as illustrative guides, seamlessly connecting theoretical principles with tangible scenarios. Whether it's the precision of a missile guidance system, the stability of an aircraft in flight, or the intricacies of a robotic manufacturing arm, control systems engineering is omnipresent. By understanding these applications, aspiring engineers can appreciate the relevance and impact of control systems in shaping technological advancements across various industries.
Furthermore, the scope of control systems engineering extends beyond the mechanical components, encompassing electrical, electronic, and software elements. This interdisciplinary nature emphasizes the need for a holistic understanding, where mechanical engineers collaborate with counterparts from diverse fields to create seamless and efficient systems. In essence, control systems engineering acts as a unifying force, harmonizing the various disciplines within the broader umbrella of engineering.
As students embark on the journey of comprehending control systems engineering, they are not merely delving into theoretical frameworks but are unlocking the door to innovation and problem-solving. The ability to manipulate and optimize dynamic systems empowers engineers to design solutions that respond intelligently to changing conditions, enhancing efficiency, safety, and performance. The mastery of control systems engineering equips individuals with a skill set that transcends academia, proving invaluable in the professional landscape.
In conclusion, understanding control systems engineering is tantamount to deciphering the language through which engineers communicate with machines, imparting precision and intelligence to their functionalities. From the foundational principles of feedback, stability, and system dynamics to the real-world applications that underscore their significance, this discipline forms the backbone of technological progress. Aspiring engineers navigating this realm embark on a transformative journey that not only enriches their academic pursuits but also prepares them to contribute meaningfully to the ever-evolving landscape of mechanical engineering.
Definition and Scope.
Control Systems Engineering, within the expansive field of mechanical engineering, revolves around the systematic design, analysis, and optimization of systems to ensure they behave in a predictable and desired manner. At its core, it is the discipline responsible for shaping the dynamics of systems through the implementation of control mechanisms. A control system, in its simplest form, consists of components that manipulate the input to a system, monitor the output, and employ feedback to regulate and maintain desired performance.
The scope of control systems engineering is both broad and pervasive, infiltrating an array of industries and technologies. From aerospace and automotive systems to manufacturing processes and robotics, the principles of control systems govern the intricate dance of variables, guiding the behavior of complex systems. These systems can range from the stability control in an automobile to the precision control of a robotic arm in a manufacturing facility. Understanding the scope involves recognizing the ubiquity of control systems in everyday life and appreciating their transformative impact on technology.
In practice, control systems extend beyond the traditional mechanical realm, embracing electrical, electronic, and software components. This interdisciplinary nature emphasizes the need for engineers versed in control systems to collaborate seamlessly across various domains. As technology continues to evolve, the scope of control systems engineering expands, with applications reaching into fields like bioengineering, environmental control, and even financial systems.
The design and implementation of control systems entail a comprehensive understanding of mathematical modeling, dynamic system analysis, and the selection of appropriate control strategies. Engineers in this field are tasked with creating systems that respond to changing conditions intelligently, ensuring stability, accuracy, and efficiency. The continual evolution of technology and the increasing complexity of systems underscore the relevance and perpetual growth of control systems engineering, making it a cornerstone in the advancement of modern engineering practices.
Key Concepts.
Key concepts in control systems engineering form the bedrock upon which the discipline builds its understanding and application. These fundamental principles are crucial for engineers to navigate the complexities of designing and optimizing systems. One primary concept is feedback, a mechanism that involves the system continuously monitoring its output and adjusting its behavior based on the observed results. Feedback is indispensable in maintaining stability, accuracy, and desired performance in dynamic systems.
Stability, another key concept, is the system's ability to return to a balanced state after encountering disturbances. A stable system ensures that deviations from the desired state are limited and transient, preventing erratic behavior. Stability analysis is a critical aspect of control systems engineering, as it lays the groundwork for predicting and controlling system responses under various conditions.
System dynamics encapsulates the study of how systems evolve over time, encompassing the understanding of transient and steady-state responses. Engineers need to grasp the dynamics of a system to predict its behavior, design effective control strategies, and ensure optimal performance. This concept becomes especially pertinent in applications where rapid changes or fluctuations are inherent.
In addition to these foundational principles, control systems engineering involves understanding and implementing control strategies. These strategies dictate how the system responds to inputs and disturbances, influencing its overall behavior. Proportional-Integral-Derivative (PID) controllers, state-space methods, and optimal control techniques are among the various strategies employed by engineers to shape the performance of control systems.
Moreover, the concept of open-loop and closed-loop systems is pivotal. An open-loop system lacks feedback, making its output independent of the current state, while a closed-loop system incorporates feedback to adjust and regulate its output. Closed-loop systems are prevalent in control systems engineering due to their ability to enhance accuracy and stability.
These key concepts collectively enable engineers to model, analyze, and design control systems effectively. By mastering feedback, stability, system dynamics, and control strategies, engineers can navigate the intricate landscape of dynamic systems, ensuring that they not only understand the theoretical underpinnings but can also apply this knowledge to solve real-world problems in diverse industries.
Common Challenges in Control Systems Assignments.
Navigating the realm of control systems assignments poses a set of challenges that often tests the mettle of aspiring engineers. One of the primary hurdles lies in the intricate process of mathematical modeling. Assignments often require students to translate physical systems into mathematical representations, a task that demands a deep understanding of both the system's behavior and the mathematical tools at hand. The challenge intensifies when dealing with complex systems where interrelated variables and dynamic behaviors need to be accurately captured. As students grapple with this, they may encounter difficulties in selecting appropriate mathematical models that balance accuracy and computational feasibility.
Controller design stands as another significant challenge in control systems assignments. Once a system is mathematically modeled, students must design controllers to regulate and manipulate the system's behavior. This involves choosing control strategies, tuning parameters, and ensuring stability. The multitude of available control strategies, such as proportional-integral-derivative (PID) controllers or state-space methods, can be overwhelming. Determining the most suitable strategy for a given system and understanding the nuances of tuning parameters require a nuanced understanding, making controller design a common stumbling block for learners.
Analysis of system response is yet another intricate facet that adds to the complexity of control systems assignments. Students are tasked with predicting and evaluating how a system will behave under different conditions. This involves assessing transient and steady-state responses, understanding how the system reacts to disturbances, and ensuring that the desired performance criteria are met. System response analysis often involves complex mathematical derivations and simulations, making it a challenging aspect for students to master.
Furthermore, the integration of software tools in control systems assignments introduces an additional layer of complexity. While software tools are indispensable for analyzing and simulating control systems, students may find it challenging to navigate these tools effectively. Understanding how to input system parameters, interpret simulation results, and troubleshoot errors can be daunting, especially for those unfamiliar with the specific software packages commonly used in control systems engineering.
The interdisciplinary nature of control systems, combining elements from mechanical, electrical, and software engineering, can be overwhelming for students. The need to integrate knowledge from different domains to solve a control systems problem adds an extra layer of complexity. This challenge requires learners to have a holistic understanding of engineering principles and the ability to apply them cohesively.
To address these challenges, students can benefit from practical examples and case studies that bridge the gap between theory and application. Additionally, hands-on experience with software tools commonly used in control systems engineering can enhance students' proficiency and confidence. Seeking assistance through tutoring, online resources, or specialized assignment help services can provide valuable guidance, ensuring that students overcome these common challenges and develop a robust foundation in control systems engineering.
Mathematical Modeling.
Mathematical modeling stands as a cornerstone in the realm of control systems engineering, representing the bridge between the physical world of dynamic systems and the abstract language of mathematics. At its essence, mathematical modeling involves translating real-world phenomena into a mathematical framework, allowing engineers to analyze, predict, and control the behavior of complex systems. In the context of control systems assignments, mastering mathematical modeling is pivotal as it forms the initial step in understanding and addressing dynamic systems.
The process of mathematical modeling begins with a comprehensive understanding of the physical system under consideration. Engineers must identify the relevant variables, parameters, and relationships governing the system's dynamics. This involves delving into the underlying physics, mechanics, or thermodynamics that dictate how the system responds to inputs and disturbances. Whether it's a mechanical system with moving parts, an electrical circuit, or a chemical process, the intricacies of the system must be dissected and translated into mathematical expressions.
Once the system's characteristics are identified, mathematical equations are formulated to represent its behavior. Differential equations, integral equations, or a combination of both are commonly employed, capturing the rates of change and interactions within the system. These equations are often nonlinear and may involve complex dynamics, requiring a nuanced understanding of mathematical techniques and tools.
The challenge in mathematical modeling for control systems assignments lies in finding a balance between simplicity and accuracy. While overly simplistic models may fail to capture essential dynamics, overly complex models can lead to computational challenges and hinder practical utility. Engineers must make informed decisions about which aspects of the system to include in the model and which to simplify, considering the trade-offs between accuracy and computational tractability.
Simulation tools and software play a crucial role in validating and refining mathematical models. Engineers use these tools to simulate the behavior of the modeled system and compare the results with real-world observations. Iterative refinement of the model based on simulation outcomes enhances its accuracy and predictive capabilities.
In conclusion, mathematical modeling serves as the linchpin for control systems engineers, enabling them to articulate the intricate dynamics of physical systems in a language that mathematics understands. Mastering this skill empowers engineers to design effective control strategies, analyze system responses, and ultimately shape the behavior of dynamic systems. Aspiring engineers engaging in control systems assignments embark on a journey where mathematical modeling is not just a theoretical exercise but a practical tool for unraveling the complexities of the engineering world.
Controller Design.
Controller design in control systems engineering is a pivotal phase where engineers craft strategies to regulate and manipulate the behavior of dynamic systems. This process is akin to orchestrating a symphony, where the controller acts as the conductor, directing the system's responses to ensure stability, accuracy, and desired performance. Controller design is a multifaceted endeavor that involves selecting appropriate control strategies, tuning parameters, and navigating the delicate balance between responsiveness and stability.
One of the fundamental aspects of controller design is the choice of control strategy, and among the most widely used is the Proportional-Integral-Derivative (PID) controller. The PID controller adjusts the system output based on proportional, integral, and derivative terms, providing a versatile framework for regulating a wide range of systems. Engineers must understand the intricacies of each term and tailor them to suit the specific characteristics of the controlled system.
Tuning the controller parameters is a critical task in controller design, and it involves adjusting the proportional, integral, and derivative gains to achieve optimal system performance. This process is often iterative, requiring engineers to analyze the system's response to different parameter values and fine-tune them to meet desired criteria such as stability, speed of response, and minimal overshot. Achieving the right balance is essential, as overly aggressive tuning may lead to instability, while conservative tuning may result in sluggish responses.
The challenge in controller design lies in navigating the diverse array of control strategies available and understanding their applicability to different systems. While PID controllers are versatile, there are instances where more advanced control methods, such as state-space control or optimal control techniques, may be more suitable. Engineers must grasp the underlying principles of these methods and discern the most effective approach for a given application.
Controller design is not a one-size-fits-all process; it requires a tailored approach based on the unique characteristics of each system. Real-world applications demand a nuanced understanding of the system's dynamics and the ability to select or design a controller that optimally balances the competing requirements of stability, responsiveness, and robustness. Aspiring control systems engineers engage in controller design as a creative and analytical endeavor, where the artistry of system control meets the precision of engineering science.
Analysis of System Response.
The analysis of system response is a crucial phase in control systems engineering, where engineers scrutinize how a dynamic system reacts to different inputs, disturbances, and changes in operating conditions. This process is fundamental for understanding the performance and stability of controlled systems, guiding engineers in fine-tuning controllers and ensuring that the system meets specified criteria.
Transient and steady-state responses are key components of system response analysis. Transient response focuses on the system's behavior during the initial phase following an input change, providing insights into how quickly the system reaches stability. Engineers evaluate parameters such as rise time, settling time, and overshoot to quantify the system's dynamic behavior. Steady-state response, on the other hand, examines the system's behavior after it has reached a stable condition, helping engineers assess the long-term performance.
Disturbance response analysis is another critical aspect, wherein engineers' study how external disturbances impact the system. Robust control systems should exhibit resilience to disturbances, and analyzing the system's response helps engineers design controllers that mitigate the effects of external influences.
Furthermore, engineers assess the system's frequency response to understand its behavior across a range of frequencies. This involves analyzing how the system responds to sinusoidal inputs at different frequencies, providing insights into its dynamic characteristics. Frequency response analysis is particularly valuable for systems where the frequency of the input signal plays a significant role, such as in communication systems or vibration control.
The choice of control strategy and controller design significantly influences the system's response. Engineers aim to strike a balance between a fast and stable response, minimizing overshot and settling time while ensuring that the system remains robust in the face of uncertainties and disturbances.
Simulation tools play a pivotal role in system response analysis, allowing engineers to model and simulate the behavior of the controlled system under various conditions. These tools provide a virtual testing ground, enabling engineers to predict and optimize system performance before implementing changes in the real-world system.
In summary, system response analysis is a comprehensive exploration of how dynamic systems behave under different conditions. It empowers control systems engineers to fine-tune controllers, optimize performance, and ensure the stability and responsiveness of controlled systems in diverse applications. Through this analytical process, engineers can enhance the predictability and efficiency of systems, contributing to advancements in various fields, from aerospace to industrial automation.
Conclusion:
In conclusion, the multifaceted realm of control systems engineering weaves together intricate principles, from mathematical modeling to controller design and the analysis of system response. This journey through the essentials of control systems engineering has illuminated the significance of these concepts in shaping the dynamic behavior of systems across diverse industries.
Control systems engineering serves as the linchpin that connects theoretical foundations with practical applications, enabling engineers to wield mathematical precision in regulating the behavior of complex systems. The mastery of mathematical modeling empowers engineers to articulate the dynamics of physical systems in a language of equations, laying the groundwork for effective control and analysis.
Controller design emerges as a symphonic endeavor where engineers craft strategies to harmonize system responses. From the versatile Proportional-Integral-Derivative (PID) controllers to advanced methods like state-space control, the artistry lies in balancing responsiveness and stability. Tuning parameters becomes a delicate dance, requiring a nuanced touch to achieve optimal system performance.
The analysis of system response completes the triad, offering engineers a window into the dynamic behavior of controlled systems. From transient responses that unveil the system's initial behavior to steady-state analyses that assess long-term performance, engineers navigate this landscape with an aim to optimize system behavior and resilience to disturbances.
As aspiring engineers delve into control systems assignments, challenges arise – be it in the intricate process of mathematical modeling, the nuanced choices in controller design, or the comprehensive analysis of system responses. However, these challenges are not roadblocks but steppingstones to mastery, opportunities for growth, and avenues to bridge the gap between theoretical knowledge and practical application.
In the grand tapestry of technological advancement, control systems engineering emerges as a vital thread, seamlessly connecting disciplines and industries. Its impact resonates in aerospace, manufacturing, robotics, and beyond, shaping the trajectory of innovation. By understanding the essentials of control systems engineering, engineers embark on a journey where theoretical knowledge converges with practical wisdom, laying the foundation for transformative contributions to the ever-evolving landscape of engineering. Through this journey, the art and science of control systems engineering continue to unfold, beckoning aspiring engineers to unravel new complexities, overcome challenges, and contribute to the progress of technology and human ingenuity.