Chemical Thermodynamics: Principles and Applications for Material Engineering Assignments
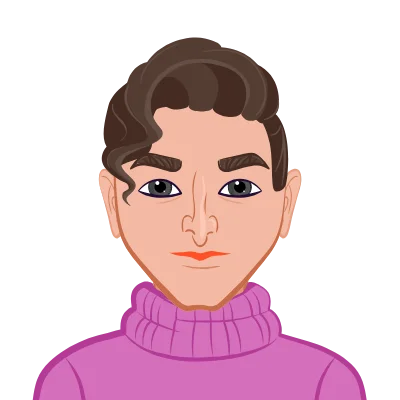
Chemical thermodynamics plays a pivotal role in the field of material engineering assignments, serving as the foundational framework that unveils the intricate behaviors of materials and their extensive applications. At its core, chemical thermodynamics is the study of the energy changes and transformations that occur during chemical reactions, providing a systematic approach to understanding the physical and chemical properties of materials. In the realm of material engineering, where the design, synthesis, and optimization of materials are central objectives, a profound grasp of chemical thermodynamics becomes indispensable.
One of the primary significances of chemical thermodynamics lies in its ability to elucidate the energetic landscape of materials. By comprehending the principles of energy, entropy, and enthalpy, material engineers gain profound insights into the fundamental forces that govern the stability, reactivity, and phase transitions of materials. This knowledge is particularly crucial when dealing with complex materials systems, where predicting and controlling the behavior of substances is paramount. Whether investigating the thermodynamic stability of alloys, the phase transitions of polymers, or the energetics of chemical reactions within materials, a thorough understanding of chemical thermodynamics empowers engineers to make informed decisions and advancements.
Furthermore, chemical thermodynamics finds extensive application in material engineering assignments, influencing every facet of the materials development process. One notable application is in the interpretation of phase diagrams, which depict the equilibrium conditions between different phases of a material as a function of temperature and pressure. Through the lens of thermodynamics, engineers can decipher the boundaries separating phases, predict the formation of specific microstructures, and optimize material processing conditions. This has profound implications for industries ranging from metallurgy to semiconductor manufacturing, where precise control over material properties is paramount.
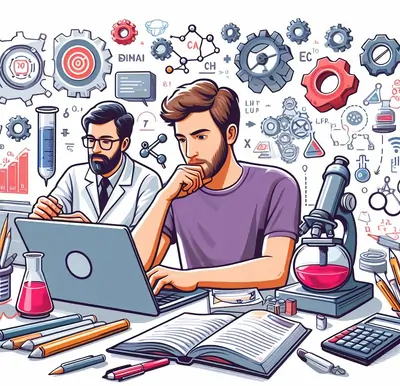
In the synthesis of materials, chemical thermodynamics guides engineers in understanding reaction kinetics and thermodynamics, ensuring the efficient and economical production of desired materials. By leveraging principles such as Gibbs Free Energy, engineers can assess the feasibility and spontaneity of reactions, thereby optimizing reaction conditions to achieve desired outcomes. This not only streamlines the synthesis process but also minimizes energy consumption and waste, aligning with the principles of sustainable and efficient material engineering. For those seeking assistance, it is important to grasp these thermodynamic principles to effectively solve your Thermodynamics assignment.
The principles of chemical thermodynamics also play a pivotal role in elucidating the stability and feasibility of materials under diverse conditions. Whether exploring the durability of materials in extreme temperatures or assessing their performance in corrosive environments, thermodynamic analyses provide a predictive toolset. Engineers can anticipate material degradation, evaluate the impact of environmental factors, and design materials with enhanced resilience. This is particularly pertinent in industries such as aerospace and renewable energy, where materials must withstand demanding conditions to ensure long-term functionality and reliability.
In conclusion, the importance of chemical thermodynamics in material engineering assignments cannot be overstated. Its role in unraveling the behavior of materials and guiding their applications is indispensable for engineers striving to innovate and optimize materials across diverse industries. By embracing the principles of chemical thermodynamics, material engineers embark on a journey of discovery and application, unlocking the full potential of materials to meet the challenges of the present and future.
Overview of Chemical Thermodynamics:
Chemical thermodynamics is a branch of physical chemistry that systematically studies the energy transformations and relationships within chemical systems. It provides a theoretical framework for understanding and predicting the behavior of materials and reactions, making it an indispensable tool in the realm of material engineering. The scope of chemical thermodynamics in material engineering is vast, encompassing the design, synthesis, and optimization of materials by delving into the energetic principles that govern their stability, reactivity, and phase transitions.
At the heart of chemical thermodynamics are fundamental concepts that form the basis for understanding the energetic aspects of materials. Energy, the first and foremost concept, is a key player in material science. Whether in the form of heat, work, or internal energy, energy changes accompany chemical reactions and transformations in materials. Engineers leverage this understanding to manipulate and control the energy states of materials, influencing their properties and performance. In material synthesis, for example, the input or release of energy during reactions is carefully considered to optimize reaction conditions and enhance the efficiency of the process.
Entropy, another crucial concept, represents the measure of disorder or randomness in a system. In material engineering, entropy is pivotal in elucidating phase transitions and the arrangement of atoms or molecules within materials. The study of entropy allows engineers to predict and control the structural changes in materials, such as the transition from solid to liquid states. This is particularly relevant in the manufacturing of alloys and polymers, where precise control over the microstructure is essential for achieving desired material properties.
Enthalpy, the third fundamental concept, combines the internal energy of a system with the product of pressure and volume. It is a measure of the heat content of a material, and its role in material science is multifaceted. Enthalpy changes are central to understanding the heat absorbed or released during chemical reactions, providing insights into reaction thermodynamics. This knowledge is crucial for engineers seeking to optimize reaction conditions, control material synthesis, and design processes with minimal energy input. Enthalpy considerations also play a vital role in material stability studies, where the ability of materials to withstand changes in temperature and pressure is a critical aspect of their performance.
In the context of material engineering, the scope of chemical thermodynamics extends to the interpretation of phase diagrams, which graphically represent the equilibrium conditions between different phases of a material. Engineers utilize thermodynamic principles to analyze these diagrams, predicting phase transitions and optimizing processing conditions. This is integral to material design, as the ability to tailor material properties through phase control is essential for meeting specific performance requirements in industries ranging from electronics to construction.
In conclusion, chemical thermodynamics forms the backbone of material engineering by providing a comprehensive framework for understanding the energetic aspects of materials. Its fundamental concepts of energy, entropy, and enthalpy are essential tools that empower engineers to predict, manipulate, and optimize the behavior of materials. Through the application of these principles, material scientists and engineers can innovate and design materials with tailored properties, meeting the ever-evolving demands of diverse industries.
Applications in Material Engineering:
Chemical thermodynamics, with its foundational principles, finds diverse and crucial applications in material engineering, playing a pivotal role in understanding, designing, and optimizing materials for various applications. One prominent application lies in the interpretation of phase diagrams, which are graphical representations illustrating the equilibrium conditions between different phases of a material under varying temperature and pressure. Engineers extensively use chemical thermodynamics to analyze and interpret phase diagrams, providing invaluable insights into the phase transitions and stability of materials. For example, in metallurgy, understanding the phase diagram of an alloy is essential for controlling the microstructure and mechanical properties of the final material. This application is not limited to metals; polymers, ceramics, and composite materials also benefit from phase diagram analysis, allowing engineers to tailor their properties through precise control of processing conditions.
Another critical application of chemical thermodynamics in material engineering is in the realm of reaction kinetics and thermodynamics during material synthesis. Chemical reactions within materials are governed by both kinetic and thermodynamic considerations. Thermodynamics offers insights into the feasibility and spontaneity of reactions, guiding engineers in selecting reaction pathways that lead to desired products. By analyzing thermodynamic properties such as Gibbs Free Energy, material scientists can predict whether a reaction is energetically favorable and determine the conditions under which it will proceed. This understanding is crucial in optimizing material synthesis processes, ensuring efficient and economical production. Whether synthesizing polymers, ceramics, or advanced composites, the integration of reaction kinetics and thermodynamics facilitates the development of materials with tailored properties and optimized performance.
Stability and feasibility studies represent yet another vital application of chemical thermodynamics in material engineering. Engineers systematically assess the stability of materials under different environmental conditions, such as temperature, pressure, and corrosive atmospheres. By conducting stability studies, they can predict the long-term behavior of materials, addressing concerns related to degradation, wear, and corrosion. Thermodynamic analyses enable the identification of stable phases and conditions, assisting in the design of materials with enhanced durability and reliability. In industries like aerospace, where materials face extreme conditions, stability studies are instrumental in ensuring the structural integrity and performance of materials over their operational lifetimes.
Furthermore, chemical thermodynamics is indispensable in feasibility studies for various materials, guiding engineers in the selection and design of materials for specific applications. This involves evaluating the thermodynamic compatibility of materials in a given environment, considering factors such as temperature, pressure, and chemical exposure. For instance, in the development of materials for energy storage applications, engineers assess the feasibility of reactions within battery materials, ensuring optimal performance and longevity. By understanding the thermodynamic landscape, they can predict the electrochemical behavior of materials and design energy storage systems with high efficiency and reliability.
In conclusion, the applications of chemical thermodynamics in material engineering are vast and far-reaching. From interpreting phase diagrams to optimizing material synthesis processes and conducting stability and feasibility studies, the principles of thermodynamics serve as indispensable tools for material scientists and engineers. Through the integration of these applications, the field continues to advance, enabling the design and development of innovative materials that meet the evolving needs of industries and technologies.
Key Principles in Material Engineering Assignments:
In the realm of material engineering assignments, a comprehensive understanding of the key principles of chemical thermodynamics is essential for tackling complex challenges and optimizing material design. One of the central principles that holds significant relevance is Gibbs Free Energy, a thermodynamic potential that combines enthalpy and entropy. The Gibbs Free Energy equation, ΔG = ΔH - TΔS, serves as a critical tool in predicting the spontaneity and feasibility of chemical reactions within materials. For material scientists and engineers, interpreting the Gibbs Free Energy change provides insights into the direction of a reaction and the potential for work to be done. A negative ΔG indicates a spontaneous process, while a positive ΔG suggests a non-spontaneous one. In material engineering assignments, the application of Gibbs Free Energy is crucial for assessing the thermodynamic favorability of reactions, guiding engineers in selecting appropriate processes for material synthesis, and optimizing conditions to achieve desired material properties. The principle of Gibbs Free Energy is thus indispensable for making informed decisions in the design and development of materials.
The relationship between thermodynamics and kinetics is another key principle that plays a pivotal role in material engineering assignments. While thermodynamics deals with the overall energy changes and feasibility of reactions, kinetics focuses on the rates at which these reactions occur. The interplay between thermodynamics and kinetics is vital in understanding and optimizing material processes. In material engineering assignments, it is essential to recognize that a reaction may be thermodynamically favorable, yet its kinetics may pose challenges. For instance, a reaction may have a negative Gibbs Free Energy change, indicating thermodynamic favorability, but if the activation energy barrier is high, the reaction may proceed at a slow rate. Engineers must carefully balance thermodynamics and kinetics to design efficient material synthesis processes. This requires considering factors such as temperature, pressure, and catalysts to not only drive reactions in the desired direction but also ensure practical reaction rates. The integration of thermodynamics and kinetics principles empowers material engineers to devise strategies for overcoming kinetic barriers and optimizing the overall efficiency of material processes.
Entropy changes and their impact on material transformations constitute another critical aspect of chemical thermodynamics in material engineering assignments. Entropy, often referred to as a measure of disorder or randomness, plays a crucial role in understanding phase transitions and transformations within materials. In assignments related to material engineering, engineers must grasp the concept of entropy changes during these transformations. For example, the solid-to-liquid transition involves an increase in entropy as molecules gain greater freedom of movement. Understanding entropy changes is essential in predicting and controlling the behavior of materials under different conditions. Engineers leverage entropy considerations to optimize processing parameters, ensuring the desired phase transformations and material properties. In this way, the study of entropy changes becomes an indispensable tool for material engineers seeking to manipulate and tailor the structure and properties of materials to meet specific requirements.
In conclusion, the key principles of chemical thermodynamics—Gibbs Free Energy, the relationship between thermodynamics and kinetics, and entropy changes—form the backbone of solving material engineering assignments. These principles provide a robust framework for predicting the spontaneity, rates, and transformations of reactions within materials. Mastering these principles empowers material engineers to make informed decisions in the design and optimization of materials, ensuring that they meet the desired specifications for diverse applications in industries ranging from electronics to aerospace.
Case Studies:
Chemical thermodynamics has been instrumental in shaping numerous real-world applications and innovations within the field of material engineering. One prominent example is the development of high-performance alloys for aerospace applications. The aerospace industry demands materials with exceptional strength, corrosion resistance, and durability. Applying the principles of chemical thermodynamics, engineers design alloys by carefully considering phase diagrams, Gibbs Free Energy changes, and stability criteria. For instance, the development of superalloys, such as those based on nickel and titanium, involves intricate thermodynamic analyses to predict the optimal composition for high-temperature strength and resistance to degradation. The success of these alloys in turbine engines, where extreme temperatures and mechanical stresses are prevalent, exemplifies the impactful application of chemical thermodynamics in achieving materials with superior performance.
In the realm of semiconductor manufacturing, the principles of chemical thermodynamics have been crucial in the innovation of materials for electronic devices. The optimization of thin film deposition processes, essential in the production of integrated circuits, relies on a deep understanding of thermodynamic principles. Engineers leverage thermodynamic considerations to control reaction kinetics during chemical vapor deposition (CVD) or physical vapor deposition (PVD), ensuring the formation of high-quality thin films with specific electrical and mechanical properties. This application of chemical thermodynamics has led to the continuous miniaturization of electronic components, enabling the production of faster and more energy-efficient devices. Overcoming challenges such as uniformity of film thickness and minimizing defects, engineers have refined these processes to achieve the precision required for cutting-edge semiconductor technologies.
In the field of renewable energy, the application of chemical thermodynamics is exemplified in the development of advanced materials for energy storage systems, such as lithium-ion batteries. Optimizing the electrochemical performance of battery materials involves understanding the thermodynamics of redox reactions and the stability of electrode materials. Engineers apply thermodynamic principles to select suitable electrode materials, predict cell voltages, and optimize the overall efficiency of energy storage systems. Successful innovations in battery technology, which power electric vehicles and store renewable energy, showcase the impact of chemical thermodynamics on sustainable energy solutions. Challenges related to battery degradation and limited cycle life have been addressed through material design guided by thermodynamic principles, leading to enhanced battery performance and longevity.
Despite these successes, challenges in applying chemical thermodynamics to material engineering persist. One common challenge is the need for accurate thermodynamic data, especially for novel materials or extreme conditions. Researchers and engineers continually work on improving databases and measurement techniques to enhance the precision of thermodynamic predictions. Additionally, the complexity of multi-component systems and the intricate interplay between thermodynamics and kinetics pose ongoing challenges. Overcoming these challenges requires interdisciplinary collaboration, advanced modeling techniques, and experimental validation.
In conclusion, the principles of chemical thermodynamics have been instrumental in driving successful applications and innovations in material engineering across various industries. From aerospace alloys to semiconductor technologies and renewable energy storage, the impact of thermodynamics on material design is profound. Engineers have overcome challenges by refining processes, leveraging advanced modeling tools, and advancing measurement techniques. As the field continues to evolve, the principles of chemical thermodynamics will undoubtedly remain indispensable in the quest for novel materials with tailored properties and enhanced performance.
Conclusion:
In summary, the blog post has delved into the critical role of chemical thermodynamics in the field of material engineering assignments. The exploration began with an introduction to the importance of chemical thermodynamics, emphasizing its role in unraveling the behaviors of materials and its widespread applications. The discussion then progressed to specific applications of chemical thermodynamics, highlighting its significance in interpreting phase diagrams, guiding reaction kinetics and thermodynamics in material synthesis, and conducting stability and feasibility studies for various materials. These applications were illustrated with real-world examples, showcasing how the principles of chemical thermodynamics have been successfully applied in industries such as aerospace, semiconductor manufacturing, and renewable energy.
The blog post further elucidated the key principles of chemical thermodynamics essential for solving material engineering assignments. The focus was on Gibbs Free Energy and its significance in predicting the spontaneity and feasibility of reactions, the relationship between thermodynamics and kinetics in balancing the overall energy changes and reaction rates, and the impact of entropy changes on material transformations. Each principle was discussed in the context of its practical application, underlining the importance of these concepts for material scientists and engineers seeking to design and optimize materials for diverse applications.
The relevance of chemical thermodynamics in material engineering assignments was reinforced by emphasizing its role as a foundational framework for understanding the energetic aspects of materials. Whether predicting phase transitions, optimizing reaction conditions, or assessing the stability of materials, the principles of chemical thermodynamics provide a systematic approach that empowers engineers to make informed decisions. The integration of thermodynamic principles is crucial for achieving desired material properties, meeting performance requirements, and driving innovations in various industries.
In conclusion, the blog post encourages readers to explore more about the topic of chemical thermodynamics and apply their knowledge in their studies. By gaining a deeper understanding of these principles, students and professionals in material engineering can enhance their problem-solving skills, make informed decisions in their projects, and contribute to the continuous advancements in material science and technology. The applications and case studies presented in the blog serve as inspiration for readers to delve into the intricacies of chemical thermodynamics, not only as a theoretical framework but as a practical tool that shapes the future of materials engineering. Ultimately, the post encourages a curiosity-driven exploration of this fundamental field and its applications, inviting readers to become active participants in the ongoing journey of discovery and innovation within material science.