Thermal Efficiency in Engine Design: Applying Thermodynamics to Improve Performance!
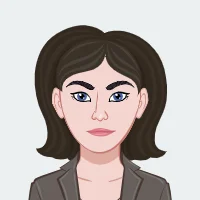
In the realm of engine design, the concept of thermal efficiency stands as a pivotal factor dictating the performance and sustainability of mechanical systems. At its core, thermal efficiency is a measure of how well an engine converts the energy from its fuel into useful work. It serves as a key metric in assessing the effectiveness of an engine in harnessing the potential energy stored within its fuel source. The importance of optimizing thermal efficiency cannot be overstated, as it directly correlates with fuel consumption, emissions, and overall operational costs. Engines operating with higher thermal efficiency not only utilize fuel more judiciously but also exhibit superior performance characteristics, such as increased power output and reduced waste heat generation. In essence, the quest for optimal thermal efficiency aligns with the broader industry goals of achieving sustainability, minimizing environmental impact, and maximizing energy utilization. Whether you're seeking help with your Thermodynamics assignment or aiming to deepen your understanding of engine performance, grasping the principles of thermal efficiency is essential for effective engineering design and sustainable technological advancement.
Understanding and improving thermal efficiency delve into the principles of thermodynamics, a field that underpins the very fabric of energy conversion within engines. Thermodynamics, in the context of engine design, provides the theoretical framework for comprehending the intricate processes involved in the transformation of thermal energy into mechanical work. The laws of thermodynamics, notably the first and second laws, guide engineers in navigating the challenges and constraints inherent in the energy conversion process. The first law, or the law of conservation of energy, asserts that energy cannot be created or destroyed but can only change forms. This principle underscores the necessity of efficient energy utilization in engines. Meanwhile, the second law introduces the concept of entropy, encapsulating the irreversibility and inherent limitations of energy conversion processes. Embracing these thermodynamic principles, engineers can identify opportunities for improvement, devise innovative solutions, and ultimately enhance the thermal efficiency of engines.
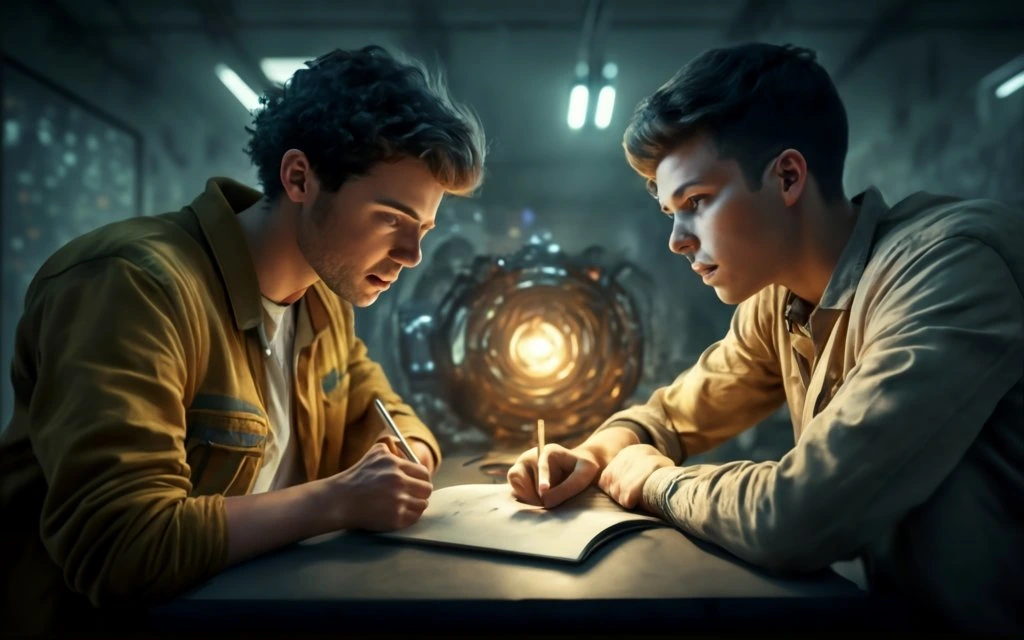
In the pursuit of heightened thermal efficiency, engineers grapple with an array of factors that impact the performance of engines. Factors such as combustion efficiency, heat transfer, and friction play pivotal roles in determining the overall efficiency of the energy conversion process. Optimizing combustion efficiency involves achieving a thorough and controlled burning of the fuel-air mixture, minimizing wasted energy in the form of unburned fuel or incomplete combustion products. Heat transfer, on the other hand, necessitates a delicate balance, ensuring that the right amount of heat is extracted from the combustion chamber to drive the engine while avoiding excessive losses to the surroundings. Additionally, addressing frictional losses within the engine components becomes crucial to maximizing thermal efficiency. These factors collectively underscore the intricate nature of thermal efficiency optimization, demanding a multidimensional approach grounded in the principles of thermodynamics.
In conclusion, the concept of thermal efficiency within engine design emerges as a linchpin for achieving sustainable and high-performance mechanical systems. By delving into the principles of thermodynamics, engineers gain profound insights into the underlying processes governing energy conversion. The relentless pursuit of optimal thermal efficiency not only aligns with industry imperatives for sustainability but also propels advancements in technology, pushing the boundaries of what is achievable in the realm of engine design. In essence, the quest for enhanced thermal efficiency epitomizes the intersection of theoretical understanding and practical application, shaping the future of efficient and eco-friendly propulsion systems.
Understanding Thermal Efficiency:
Thermal efficiency is a critical metric in the realm of mechanical engineering, serving as a key indicator of how effectively an engine converts input energy into useful work. In essence, it represents the ratio of the work output of a system to the heat input, reflecting the engine's ability to transform thermal energy into mechanical energy. Significantly, thermal efficiency is pivotal in determining the overall performance and sustainability of an engine, playing a crucial role in the design, optimization, and evaluation of mechanical systems.
To comprehend the significance of thermal efficiency, one must delve into the basic thermodynamic principles governing energy conversion in engines. Thermodynamics, a fundamental branch of physics, explores the relationships between heat, work, and energy transfer. In the context of engines, thermodynamics elucidates the processes involved in converting fuel's chemical energy into mechanical work. The first law of thermodynamics, also known as the law of energy conservation, establishes that energy cannot be created or destroyed, only converted between forms. Consequently, engines operate within this framework, with heat input from fuel leading to an increase in internal energy and subsequent conversion to mechanical work.
The concept of thermal efficiency becomes particularly pertinent when comparing the ideal and actual performance of engines. The ideal thermal efficiency, dictated by Carnot's theorem, establishes an upper limit on efficiency based on the temperature difference between the heat source and sink. However, real-world engines inevitably face limitations due to factors such as friction, heat dissipation, and irreversibilities in the combustion process. As a result, actual thermal efficiency is always lower than the ideal, with the ratio of actual to ideal efficiency often expressed as a percentage termed as the second law efficiency. Recognizing and mitigating these losses is paramount in the pursuit of enhancing overall engine efficiency.
In conclusion, thermal efficiency is a cornerstone in the field of mechanical engineering, embodying the effectiveness of energy conversion in engines. The understanding of thermodynamic principles underpinning this efficiency is indispensable for engineers seeking to optimize and innovate in the realm of engine design. By comprehending the delicate balance between ideal and actual thermal efficiency, engineers can develop strategies to enhance performance, reduce energy losses, and propel advancements in the efficiency and sustainability of mechanical systems.
Factors Affecting Thermal Efficiency:
In the intricate realm of engine design, achieving optimal thermal efficiency involves a nuanced understanding of several interrelated factors that significantly influence performance. One pivotal aspect is combustion efficiency, the process by which fuel is efficiently converted into useful work. Efficient combustion reduces waste and maximizes the extraction of energy from the fuel, directly impacting the thermal efficiency of the engine. However, achieving high combustion efficiency is a delicate balance, as factors such as air-fuel ratio, ignition timing, and combustion chamber design must be meticulously tuned to prevent incomplete combustion or excessive heat losses.
Heat transfer within the engine constitutes another critical determinant of thermal efficiency. The challenge lies in managing heat effectively to harness it for useful work while minimizing losses. Efficient heat transfer involves optimizing the design of cooling systems, ensuring proper insulation, and mitigating thermal gradients. Excessive heat loss to the surroundings can diminish thermal efficiency, emphasizing the need for innovative materials and engineering solutions that strike a delicate equilibrium between heat retention and dissipation.
Friction, though often considered an inherent drawback, plays a crucial role in engine design. While friction between moving parts is inevitable, mitigating its impact is imperative for improving overall efficiency. Lubrication technologies and materials with reduced friction coefficients are continually researched and implemented to minimize energy losses attributed to friction. However, this pursuit of reduced friction encounters trade-offs, as some high-performance engines may sacrifice certain degrees of friction for enhanced power output.
Navigating these factors involves a series of challenges and trade-offs. For instance, achieving higher combustion efficiency might demand a leaner air-fuel mixture, but this approach can lead to increased combustion temperatures and the formation of nitrogen oxides (NOx), presenting an environmental challenge. Similarly, optimizing heat transfer may require intricate cooling systems, yet adding complexity can compromise the weight and simplicity of the engine design. Moreover, reducing friction often involves the use of specialized lubricants, which might introduce additional complexities in terms of maintenance and environmental impact.
In the pursuit of enhanced thermal efficiency, engineers face a perpetual juggling act, where improvements in one aspect may necessitate compromises in another. The optimization process is a delicate dance, and each engine design represents a unique solution tailored to balance the intricate interplay of combustion efficiency, heat transfer, and friction. As technology advances, the industry continues to grapple with these challenges, driven by the overarching goal of pushing the boundaries of thermal efficiency while addressing environmental concerns and consumer demands for reliable, high-performance engines.
Strategies to Improve Thermal Efficiency:
In the relentless pursuit of enhancing thermal efficiency in internal combustion engines, engineers have harnessed a myriad of innovative strategies and cutting-edge technologies to propel the field of engine design forward. Among the foremost advancements, variable valve timing (VVT), turbocharging, and direct injection stand out as transformative contributors to the quest for improved thermal efficiency.
Variable valve timing, a technological marvel, enables precise control over the timing of the opening and closing of engine valves. By dynamically adjusting the valve timing based on engine speed, load, and other factors, VVT optimizes combustion efficiency. This results in better utilization of fuel and air, ultimately leading to improved thermal efficiency. The ability to fine-tune valve operations enhances overall engine performance, striking an optimal balance between power output and fuel consumption.
Turbocharging, another game-changing innovation, exploits exhaust gases to force more air into the engine's combustion chamber. This compressed air allows for a more substantial fuel charge, promoting more vigorous combustion. The net effect is an increase in power without a corresponding spike in fuel consumption. By capitalizing on otherwise wasted energy, turbocharging substantially boosts thermal efficiency, making engines more environmentally friendly and economically viable.
Direct injection represents a paradigm shift in fuel delivery mechanisms, offering superior control over the injection process. Unlike traditional port fuel injection, direct injection introduces fuel directly into the combustion chamber at high pressure. This precision facilitates better mixing of fuel and air, resulting in more efficient combustion. The enhanced control over the combustion process not only enhances power output but, critically, contributes to heightened thermal efficiency by minimizing energy losses associated with incomplete combustion.
Collectively, these advancements synergistically contribute to elevating thermal efficiency in engine design. Variable valve timing, turbocharging, and direct injection work in tandem to address the intricacies of combustion dynamics, reducing waste and optimizing energy utilization. The integration of these technologies embodies a holistic approach to enhancing thermal efficiency, demonstrating a commitment to sustainable engineering practices. As the automotive industry pivots toward more environmentally conscious solutions, these innovations underscore the potential for achieving higher efficiency standards without compromising performance. In the evolving landscape of engine design, the adoption of these technologies heralds a new era where optimal thermal efficiency is not merely an aspiration but a tangible reality, shaping the future of transportation and mechanical engineering.
Case Studies: Successful Applications of Thermodynamics:
In the realm of mechanical engineering, several groundbreaking engine designs and projects have harnessed the principles of thermodynamics to achieve remarkable gains in thermal efficiency. One notable example is the development of the Toyota Prius, a pioneer in the hybrid automotive sector. The Prius incorporates a sophisticated thermodynamic system that seamlessly combines a traditional internal combustion engine with an electric motor. Through the use of regenerative braking and advanced energy management systems, the Prius optimizes thermodynamic efficiency by recovering and reusing energy that would otherwise be dissipated as waste heat. This innovative approach has propelled the Prius to the forefront of fuel-efficient vehicles, showcasing the tangible benefits of applying thermodynamics to automotive design.
Another exemplary case is the development of aircraft engines, where thermodynamics plays a crucial role in enhancing efficiency. The Pratt & Whitney Geared Turbofan (GTF) engine stands out as a paradigm of thermodynamic innovation in aviation. By employing a geared architecture, the GTF optimizes the engine's operation at different speeds, reducing energy losses and improving overall thermal efficiency. This groundbreaking design has not only led to significant fuel savings but has also reduced emissions and enhanced the sustainability of air travel. The success of the GTF engine underscores the transformative impact of thermodynamics on the aviation industry.
In the power generation sector, the combined cycle gas turbine (CCGT) power plants exemplify the application of thermodynamics to achieve higher thermal efficiency. These plants integrate both gas and steam turbines, allowing them to harness the waste heat from the gas turbine to produce additional power through the steam turbine. This combined approach significantly boosts overall efficiency, making CCGT plants a preferred choice for large-scale power generation. The integration of thermodynamic principles in CCGT plants has not only increased energy output but has also contributed to the reduction of greenhouse gas emissions, marking a crucial stride towards sustainable energy production.
In conclusion, the tangible successes of projects like the Toyota Prius, Pratt & Whitney GTF engine, and CCGT power plants demonstrate the transformative power of applying thermodynamics to improve thermal efficiency. These real-world examples showcase how innovative engineering solutions, inspired by the principles of thermodynamics, have not only optimized energy utilization but have also propelled industries towards a more sustainable and environmentally conscious future. As technology continues to advance, these success stories serve as beacons, guiding the way for future endeavors to further unlock the potential of thermodynamics in enhancing efficiency across various engineering domains.
Conclusion:
In the blog post titled "Thermal Efficiency in Engine Design: Applying Thermodynamics to Improve Performance," we delved into the critical aspects of thermal efficiency and its profound impact on engine design within the realm of mechanical engineering. The exploration commenced by establishing a fundamental understanding of thermal efficiency, elucidating its significance in the broader context of mechanical engineering, and spotlighting the indispensable role played by thermodynamics in comprehending and enhancing engine performance.
The discourse ventured into the intricate factors influencing thermal efficiency, dissecting the complexities of combustion efficiency, heat transfer, and friction. An examination of these elements revealed the intricate interplay that engineers must navigate to optimize overall efficiency, with an acknowledgment of the challenges and trade-offs inherent in the pursuit of this goal. Moving forward, the blog elucidated various strategies employed to elevate thermal efficiency, spotlighting advanced technologies like variable valve timing, turbocharging, and direct injection as pivotal contributors to performance enhancement.
Drawing upon real-world applications, the blog presented compelling case studies that showcased the successful application of thermodynamics in achieving remarkable gains in thermal efficiency. These examples underscored the tangible impact of integrating theoretical principles into practical engineering solutions. Furthermore, a forward-looking lens was applied to scrutinize future trends and innovations in engine design, emphasizing emerging technologies such as electric and hybrid propulsion systems, alternative fuels, and sustainable engineering practices.
A resounding theme throughout the blog was the perpetual need for research and development in the pursuit of higher thermal efficiency. The importance of staying abreast of advancements in engine design and thermodynamics was underscored as an ongoing commitment essential for engineers and enthusiasts alike. Recognizing that the landscape of mechanical engineering is dynamic, readers were encouraged to actively engage with evolving developments, fostering a mindset of continual learning and adaptation.
In conclusion, the blog served as a comprehensive exploration of the intricacies surrounding thermal efficiency in engine design, offering valuable insights into its theoretical foundations, practical applications, and future trajectories. Emphasizing the crucial role of research and development, the narrative urged readers to remain vigilant and informed, positioning themselves at the forefront of the ever-evolving field of mechanical engineering, where the pursuit of higher thermal efficiency continues to drive innovation and progress.