System Optimization Techniques for Mechanical Engineering Students' Assignments
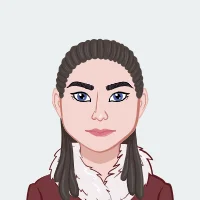
System optimization plays a pivotal role in the realm of mechanical engineering assignments, serving as a linchpin that bridges theoretical knowledge with real-world applications. At its core, optimization is the systematic process of refining and enhancing mechanical systems to achieve the best possible performance within defined constraints. In the academic arena, mastering system optimization techniques is not merely an academic pursuit; it is a gateway to elevating the quality of mechanical engineering assignments to new heights. Whether you're a student seeking help with your mechanical engineering assignment or aiming to delve deeper into the intricacies of system optimization, our comprehensive insights are designed to support your academic journey.
The significance of system optimization in mechanical engineering assignments lies in its ability to translate theoretical concepts into practical solutions. In a field where precision, efficiency, and functionality are paramount, optimization techniques act as a compass, guiding students through the intricate maze of design and analysis. By incorporating these techniques, students can engineer solutions that not only meet the specified requirements but do so with an unparalleled level of effectiveness.
One of the key aspects underscoring the importance of system optimization is its role in fostering a deep understanding of the intricate interplay between variables in a mechanical system. Assignments often present students with complex scenarios where multiple parameters influence the overall performance of a system. Mastery of optimization techniques empowers students to dissect these scenarios, identify critical factors, and manipulate variables to achieve optimal outcomes. This not only hones analytical skills but also instills a problem-solving mindset that is foundational to success in the field.
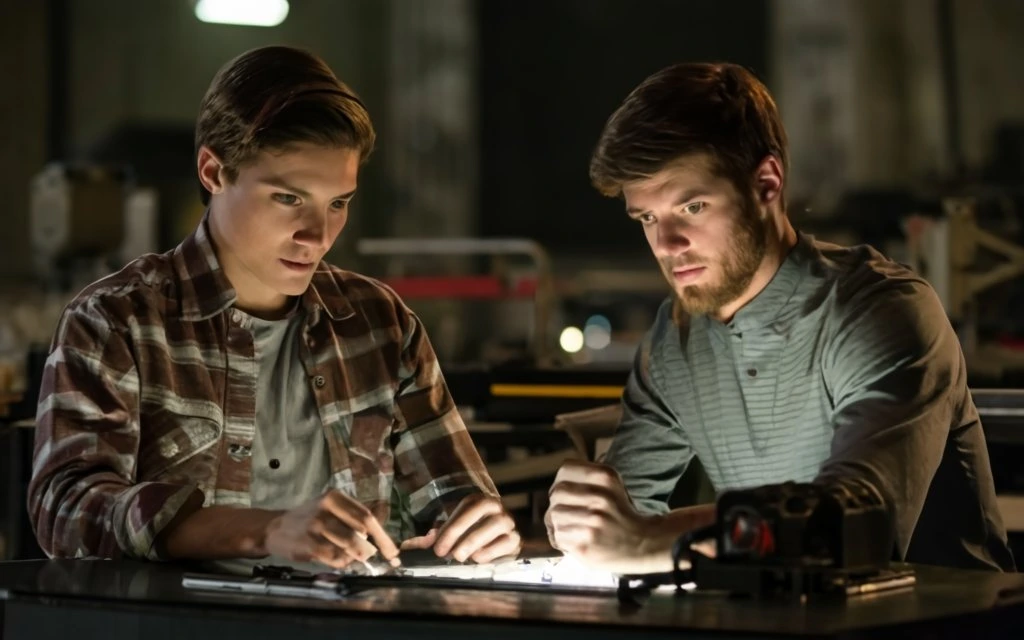
Moreover, system optimization acts as a catalyst for innovation in mechanical engineering assignments. It encourages students to move beyond conventional approaches, pushing the boundaries of what is possible. By embracing optimization, assignments transform from routine tasks into dynamic challenges that inspire creativity and ingenuity. Students learn to navigate the delicate balance between conflicting design objectives, finding ingenious solutions that maximize performance while adhering to constraints. This infusion of creativity not only enriches the learning experience but also prepares students for the multifaceted demands of the professional landscape.
The mastery of optimization techniques also imparts a practical dimension to mechanical engineering assignments. In the real world, engineering solutions are not judged solely on theoretical merit but on their tangible impact and efficiency. Optimization provides students with the tools to bridge the gap between theory and application, ensuring that their assignments reflect a pragmatic understanding of real-world constraints. This practical acumen is an asset, as it equips students with the skills needed to navigate the complexities of the industry upon graduation.
Furthermore, the application of system optimization enhances the overall quality of mechanical engineering assignments by fostering a culture of continuous improvement. Assignments cease to be isolated tasks and transform into iterative processes where each iteration builds upon the lessons learned from the previous one. Optimization encourages students to critically evaluate their designs, identify shortcomings, and iteratively refine their solutions. This iterative approach mirrors industry practices, where constant refinement and optimization are integral to the evolution of engineering solutions.
In conclusion, the importance of system optimization in mechanical engineering assignments cannot be overstated. It is the cornerstone that transforms theoretical knowledge into practical proficiency, fostering a holistic understanding of mechanical systems. The mastery of optimization techniques not only elevates the quality of assignments but also equips students with the skills and mindset necessary for success in the dynamic field of mechanical engineering. As students delve into the intricacies of optimization, they not only enhance their academic prowess but also embark on a journey that prepares them to be innovative, practical, and resilient engineers in the professional arena.
Understanding System Optimization.
In the dynamic realm of mechanical engineering, system optimization emerges as a cornerstone, playing a pivotal role in shaping the landscape of design and analysis. At its essence, system optimization refers to the meticulous process of refining and fine-tuning mechanical systems to achieve the most efficient and effective performance possible, given a set of constraints. In the context of mechanical engineering, these systems can encompass a broad spectrum, ranging from intricate machinery and structural components to thermal and fluid systems. Optimization, in this sense, is the art and science of navigating the multifaceted design space to uncover solutions that not only meet specified criteria but do so with optimal efficiency.
The relevance of optimization in the design and analysis of mechanical systems becomes apparent when one considers the intricate web of factors influencing the performance of these systems. Mechanical engineering is inherently interdisciplinary, with designs often involving a delicate balance between competing objectives, such as maximizing efficiency, minimizing costs, and adhering to safety regulations. Optimization serves as a guiding principle that enables engineers to navigate this complex terrain systematically. By employing mathematical models, algorithms, and simulation tools, engineers can iteratively refine their designs, ensuring that the final solution represents the pinnacle of performance within the given constraints.
In the design phase, optimization becomes a linchpin for achieving innovation and efficiency. Engineers are faced with the challenge of creating systems that not only fulfill their intended purpose but do so with the greatest possible efficacy. Optimization allows for the exploration of a vast design space, where variables can be adjusted, and parameters fine-tuned to discover configurations that outperform others. This process is particularly crucial in an era where sustainability and resource efficiency are paramount. Optimization empowers engineers to craft solutions that not only meet functional requirements but also align with the broader goals of sustainability and environmental responsibility.
Moreover, optimization plays a pivotal role in the analysis of mechanical systems. In a discipline where performance metrics are integral to success, engineers must not only design functional systems but also ensure that these systems operate at their peak potential. Through the application of optimization techniques, engineers can analyze and enhance the performance of existing systems, identifying areas for improvement and fine-tuning parameters to maximize efficiency. This iterative process of analysis and optimization is a hallmark of engineering practice, mirroring the cyclical nature of improvement and innovation that defines the field.
In the ever-evolving landscape of mechanical engineering, where technological advancements continuously raise the bar for performance and efficiency, the relevance of system optimization becomes even more pronounced. As the complexity of mechanical systems increases, so does the need for systematic approaches to design and analysis. Optimization provides the methodology to navigate this complexity, offering engineers a structured framework to uncover optimal solutions. It is the catalyst for pushing the boundaries of what is possible, ensuring that mechanical systems not only meet expectations but exceed them, setting new standards for efficiency, innovation, and excellence in the field.
Key Optimization Techniques.
Optimization techniques in the realm of mechanical engineering constitute a diverse toolkit of methodologies and approaches aimed at refining and enhancing the performance of systems. These techniques are fundamental to the design, analysis, and improvement of mechanical systems, providing engineers with systematic ways to achieve optimal solutions within specified constraints. Several key optimization techniques stand out in the field, each playing a distinct role in shaping the efficiency and functionality of mechanical systems.
Optimization techniques in mechanical engineering represent a diverse and indispensable toolkit, steering the course of design and analysis toward efficiency and excellence. At the core of these techniques is mathematical modeling, where engineers formulate intricate mathematical representations of mechanical systems. This process allows for a systematic exploration of design alternatives, enabling the identification of configurations that achieve optimal performance. Complementing this, simulation tools provide a virtual testing ground, allowing engineers to analyze system behavior under various conditions. Simulation facilitates the identification of areas for improvement and fine-tuning, reducing the need for extensive physical prototypes.
Parameter optimization stands as a fundamental technique, involving the meticulous adjustment of system parameters to maximize desired outcomes. This nuanced process demands a deep understanding of a system's intricacies, challenging engineers to balance competing objectives and refine their problem-solving skills. Sensitivity analysis adds a layer of insight by gauging the robustness of a system to parameter variations, guiding optimization efforts toward the most impactful variables. Real-world case studies bring a practical dimension, illustrating how optimization has been instrumental in overcoming engineering challenges across diverse applications.
For students, navigating mechanical engineering assignments requires mastery of these optimization techniques. It involves not only the formulation of accurate mathematical models but also the application of simulation tools, parameter adjustments, and sensitivity analyses. Emphasizing a systematic, iterative approach, interdisciplinary thinking, and a curiosity-driven mindset is crucial. Optimization techniques, when applied strategically, transform assignments into iterative processes of continuous improvement, preparing students for the complexities of real-world engineering. As beacons of innovation, these techniques bridge the gap between theory and application, guiding engineers to solutions that are not just functional but optimal, shaping the trajectory of mechanical engineering toward new frontiers of efficiency and excellence.
Optimization techniques in the field of mechanical engineering constitute a multifaceted toolkit that empowers engineers to refine, enhance, and perfect the performance of systems. These techniques, ranging from mathematical modeling to simulation and sensitivity analysis, serve as guiding principles in the pursuit of efficiency, functionality, and innovation.
Mathematical Modeling:
Mathematical modeling stands as a cornerstone in the arsenal of optimization techniques within the realm of mechanical engineering, providing engineers with a powerful tool to conceptualize, analyze, and optimize complex systems. At its essence, mathematical modeling involves the translation of real-world phenomena into quantifiable mathematical expressions, allowing for a systematic representation of the relationships between variables in a mechanical system. This process facilitates a deep understanding of the system's behavior, enabling engineers to predict its performance under various conditions and iterate on design parameters.
In the context of mechanical engineering assignments, the significance of mathematical modeling cannot be overstated. Students are tasked with grappling with the intricacies of mechanical systems, and the ability to create accurate mathematical models is fundamental to their success. Whether it be structural mechanics, fluid dynamics, or thermal analysis, the precision afforded by mathematical models empowers students to dissect complex problems systematically. By formulating equations and algorithms that encapsulate the underlying physics of a system, students gain a quantitative grasp of its behavior, laying the foundation for subsequent optimization efforts.
Moreover, mathematical modeling serves as the launching pad for optimization endeavors. Engineers can manipulate these mathematical representations to explore different design scenarios, adjusting variables to identify configurations that yield optimal performance. This iterative process of refining and fine-tuning designs is a hallmark of engineering practice, and mathematical modeling provides the necessary framework for this exploration. It allows engineers to navigate the intricate design space, optimizing parameters to meet specific criteria and pushing the boundaries of what is achievable within the constraints of a given project.
In summary, mathematical modeling is not merely a theoretical exercise; it is a practical and indispensable tool for engineers and students alike. It bridges the gap between conceptual understanding and tangible application, fostering a systematic and analytical approach to solving engineering challenges. As a foundational element of optimization, mathematical modeling empowers individuals to unlock the full potential of mechanical systems, guiding them towards solutions that are not only functional but finely tuned for optimal performance.
Central to the arsenal of optimization techniques is mathematical modeling, an approach that involves the formulation of mathematical representations of mechanical systems. Through equations and algorithms, engineers can capture the dynamic interplay of variables within a system, translating real-world phenomena into quantifiable terms. This modeling process not only provides a conceptual framework for understanding system behavior but also lays the groundwork for optimization. Engineers can manipulate these mathematical models to explore different scenarios, adjusting variables to identify configurations that yield optimal performance.
In the realm of mechanical engineering assignments, mathematical modeling becomes a cornerstone for problem-solving. Students are tasked with understanding and articulating the intricacies of mechanical systems, and the ability to create accurate mathematical models is pivotal. Whether it involves structural analysis, fluid dynamics, or thermal systems, the precision afforded by mathematical models allows for a systematic exploration of design alternatives and the identification of optimal solutions.
Simulation and Analysis:
Simulation and analysis represent pivotal facets of the optimization toolkit in the domain of mechanical engineering, offering engineers a virtual laboratory to scrutinize, refine, and enhance the performance of intricate systems. Simulation, in this context, involves the utilization of advanced software tools to replicate the behavior of a mechanical system under diverse conditions, without the need for physical prototypes. This computational approach provides a dynamic platform for engineers to subject their designs to rigorous testing, enabling the observation of system responses, identifying potential issues, and evaluating performance metrics.
In the realm of mechanical engineering assignments, simulation is transformative. It serves as a bridge between theoretical concepts and practical application, allowing students to explore the real-world implications of their designs in a controlled digital environment. Through simulation, students can iteratively refine their models, adjusting parameters and assessing the impact of these changes on the overall system. This hands-on experience not only hones their analytical skills but also instills a pragmatic understanding of how different design choices influence the behavior and efficiency of mechanical systems.
Analysis, closely intertwined with simulation, involves a detailed examination of the data generated during simulated scenarios. Engineers dissect the results to gain insights into system performance, identify bottlenecks, and uncover areas for improvement. This analytical phase is critical in the optimization process, guiding engineers to make informed decisions about design modifications and parameter adjustments.
In educational settings, incorporating simulation tools into the curriculum equips students with a skill set that is increasingly valued in the professional landscape. It not only enhances their proficiency in using state-of-the-art software but also instills a mindset of continuous improvement. Students learn to leverage simulation not merely as a validation tool but as a proactive means of refining their designs, aligning with the iterative nature of engineering optimization.
Simulation and analysis, as integral components of the optimization toolkit, epitomize the marriage of technology and engineering acumen. They empower practitioners and students alike to navigate the complexities of mechanical systems, unraveling intricate relationships, and ensuring that designs are not only theoretically sound but practically robust. In the ever-evolving landscape of mechanical engineering, simulation and analysis stand as dynamic tools that propel the field toward innovation and excellence.
Simulation tools represent another key optimization technique, allowing engineers to subject their mathematical models to virtual testing and analysis. These tools enable the emulation of real-world conditions, providing a platform for assessing the performance of a system without the need for physical prototypes. Through simulations, engineers can observe how variations in parameters affect the overall behavior of a system, identifying areas for improvement and optimization.
In the educational landscape, introducing students to simulation tools not only enhances their understanding of theoretical concepts but also instills practical skills that are invaluable in professional practice. By engaging with simulations, students can bridge the gap between theory and application, gaining a holistic understanding of how different design choices impact the performance of a system. This hands-on experience prepares them for the challenges of real-world engineering, where the ability to analyze and optimize systems through simulation is a prized skill.
Parameter Optimization:
Parameter optimization emerges as a fundamental and intricate facet within the spectrum of mechanical engineering, where the nuanced adjustment of system parameters holds the key to unlocking optimal performance. At its essence, this technique involves a meticulous examination of the variables influencing a mechanical system, with the aim of refining these parameters to achieve the most efficient and effective outcomes. Engineers navigate a delicate balance, tweaking dimensions, material properties, or operating conditions to meet specified design objectives, whether it be maximizing efficiency, minimizing costs, or adhering to safety standards.
In the realm of mechanical engineering assignments, parameter optimization tasks students with a dynamic and iterative process. It requires a deep understanding of the system's intricacies and the interconnectedness of variables. Students embark on a journey where each adjustment triggers a cascade of effects on the overall performance of the system. This iterative refinement not only sharpens their problem-solving skills but also cultivates an appreciation for the multifaceted nature of engineering challenges. It underscores the reality that successful parameter optimization demands not just theoretical knowledge but a hands-on, trial-and-error approach.
The significance of parameter optimization is amplified in the broader context of engineering practice. In the design phase, engineers grapple with the challenge of creating systems that not only function as intended but do so with optimal efficiency. Parameter optimization becomes a strategic tool in achieving this delicate balance. It encourages engineers to think critically about the trade-offs inherent in design choices, pushing the boundaries of what is possible within the constraints of a given project.
Moreover, parameter optimization aligns with the broader industry shift towards sustainable and resource-efficient practices. Engineers are tasked not only with meeting performance criteria but also with crafting solutions that minimize environmental impact. Parameter optimization becomes a tool for achieving this balance, guiding engineers to configurations that not only enhance performance but also contribute to broader goals of sustainability.
In summary, parameter optimization in mechanical engineering epitomizes the precision and artistry required to fine-tune systems for optimal performance. It is a process that demands a profound understanding of system dynamics, an iterative mindset, and a keen awareness of the broader implications of design choices. As a fundamental optimization technique, parameter optimization not only refines mechanical systems but also shapes the mindset of engineers, preparing them for the multifaceted challenges that define the dynamic landscape of mechanical engineering.
A fundamental optimization technique revolves around the adjustment of system parameters to achieve optimal performance. In mechanical engineering assignments, this entails a meticulous examination of the factors that influence a system's behavior and the fine-tuning of these parameters to maximize desired outcomes. Whether it be adjusting the dimensions of a structural component or varying the operating parameters of a machine, parameter optimization is a nuanced process that demands a deep understanding of the system under consideration.
Students navigating mechanical engineering assignments are often tasked with optimizing parameters to meet specific design objectives. This involves a delicate balancing act, as changes to one parameter may have cascading effects on others. Through parameter optimization, students not only refine their problem-solving skills but also cultivate an appreciation for the interconnectedness of variables within a mechanical system. This iterative process of adjusting parameters, analyzing outcomes, and refining choices is emblematic of the optimization mindset.
Sensitivity Analysis:
Sensitivity analysis stands as a crucial and sophisticated optimization technique in the field of mechanical engineering, offering engineers a nuanced understanding of how variations in input parameters influence the robustness and responsiveness of a system. At its core, sensitivity analysis involves systematically perturbing input values—such as material properties, dimensions, or operating conditions—and observing the corresponding changes in the system's output. This process provides valuable insights into which parameters exert the most significant impact on the system's performance, guiding engineers towards a focused and strategic approach to optimization.
In the intricate landscape of mechanical engineering assignments, sensitivity analysis becomes a powerful tool for students navigating the complexities of system behavior. It encourages a comprehensive exploration of the design space, allowing students to discern not only the critical parameters that demand meticulous attention but also those that exhibit a degree of tolerance to variations. This awareness is instrumental in prioritizing optimization efforts, ensuring that resources are concentrated on the aspects of the system that have the most substantial influence on performance.
Sensitivity analysis also plays a pivotal role in the professional practice of engineering, where the stakes are often high, and decisions must be well-informed. By identifying parameters that significantly impact a system's output, engineers can focus their efforts on refining and optimizing those aspects, streamlining the design and analysis process. This targeted approach aligns with the industry's demand for efficiency and precision, where engineering solutions must not only meet requirements but do so in an optimized and resource-conscious manner.
Furthermore, sensitivity analysis aligns with the broader goals of sustainable engineering. Understanding which parameters have a substantial impact allows engineers to make informed decisions that minimize environmental impact and resource usage. In an era where sustainability is a paramount consideration, sensitivity analysis becomes a tool for crafting engineering solutions that are not only optimized for performance but also mindful of the ecological footprint.
In conclusion, sensitivity analysis is a sophisticated optimization technique that empowers both students and professional engineers to navigate the intricate relationships within mechanical systems. It enhances decision-making by pinpointing critical parameters, guiding the optimization process, and aligning engineering solutions with the imperatives of efficiency and sustainability. As a dynamic component of the optimization toolkit, sensitivity analysis ensures that engineering efforts are not only effective but also strategically directed, setting the stage for innovations that transcend mere functionality and embody optimized excellence.
Sensitivity analysis emerges as a crucial optimization technique, offering engineers insights into the robustness and responsiveness of a system to variations in input parameters. By systematically perturbing input values, engineers can gauge the sensitivity of the system's output, identifying parameters that exert the most significant influence on performance. This understanding is instrumental in focusing optimization efforts on the most impactful variables, streamlining the design and analysis process.
In the context of mechanical engineering assignments, sensitivity analysis equips students with a nuanced appreciation for the factors that drive system behavior. It encourages a comprehensive examination of the design space, allowing students to discern which parameters warrant meticulous attention and which exhibit a degree of tolerance. This awareness guides students in making informed decisions during the optimization process, ensuring that efforts are directed towards aspects of the system that truly influence its performance.
Conclusion:
In the intricate world of mechanical engineering assignments, the exploration of system optimization techniques serves as a guiding light, illuminating the path toward precision, efficiency, and innovation. From the foundational principles of mathematical modeling to the dynamic realms of simulation, parameter optimization, and sensitivity analysis, these techniques constitute a comprehensive toolkit that empowers students to transcend theoretical boundaries and delve into the practical intricacies of designing and analyzing mechanical systems.
As students embark on the journey of mastering these optimization techniques, they not only enhance their problem-solving skills but also cultivate a mindset of continuous improvement. The blog has delved into the significance of mathematical modeling, showcasing its role in providing a conceptual framework for understanding system behavior and laying the groundwork for subsequent optimization endeavors. Simulation and analysis emerged as dynamic companions, offering a virtual testing ground where students can iteratively refine their designs, bridging the gap between theory and application.
Parameter optimization, a fundamental technique, challenges students to navigate the delicate balance of tweaking system variables to achieve optimal outcomes. This iterative process not only sharpens their analytical prowess but also underscores the interconnectedness of design choices in the real-world engineering landscape. Sensitivity analysis, a sophisticated tool, encourages a nuanced exploration of the design space, guiding students to discern critical parameters and prioritize optimization efforts strategically.
In the broader context of professional engineering practice, these optimization techniques are not mere academic exercises; they are the catalysts for innovation and efficiency. The skills cultivated through mathematical modeling, simulation, parameter optimization, and sensitivity analysis prepare students for the multifaceted challenges of the industry, where engineering solutions must not only meet requirements but do so with optimal performance, resource efficiency, and sustainability.
As the blog concludes, it is evident that the journey through system optimization techniques equips mechanical engineering students with a holistic skill set, setting the stage for their success in academia and beyond. By embracing these techniques, students not only elevate the quality of their assignments but also embody the spirit of excellence that defines the ever-evolving landscape of mechanical engineering. The optimization journey is not just a means to an end; it is a transformative experience that propels students toward a future where innovation and efficiency converge in the creation of engineering solutions that transcend expectations.