Practical Tips for Solving Mechanical Engineering Mechanics Assignments
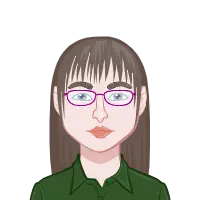
Understanding mechanics is a cornerstone of success in the field of mechanical engineering, forming the bedrock upon which a myriad of complex systems and structures are built. As students embark on their academic journey in this discipline, a profound comprehension of mechanics becomes paramount, as it not only lays the foundation for subsequent courses but also sharpens problem-solving skills essential for real-world applications. In the realm of mechanical engineering assignments, mastering mechanics is akin to possessing a set of keys that unlock the doors to a vast array of engineering challenges. If you need help with your mechanical engineering assignment, acquiring a solid grasp of these fundamental principles is crucial for tackling the intricacies of the tasks at hand.
The challenges faced by students in grappling with mechanics assignments are multifaceted and demand a nuanced approach. One of the primary hurdles lies in the intricate nature of mechanical systems, where components interact in dynamic ways. Understanding the forces at play, the equilibrium of structures, and the principles of motion can be akin to deciphering a complex puzzle for students. Furthermore, the application of abstract theoretical concepts to practical problem-solving often poses a significant challenge. The transition from theoretical knowledge to its real-world application necessitates a deep understanding of how mechanical principles manifest in tangible engineering scenarios.
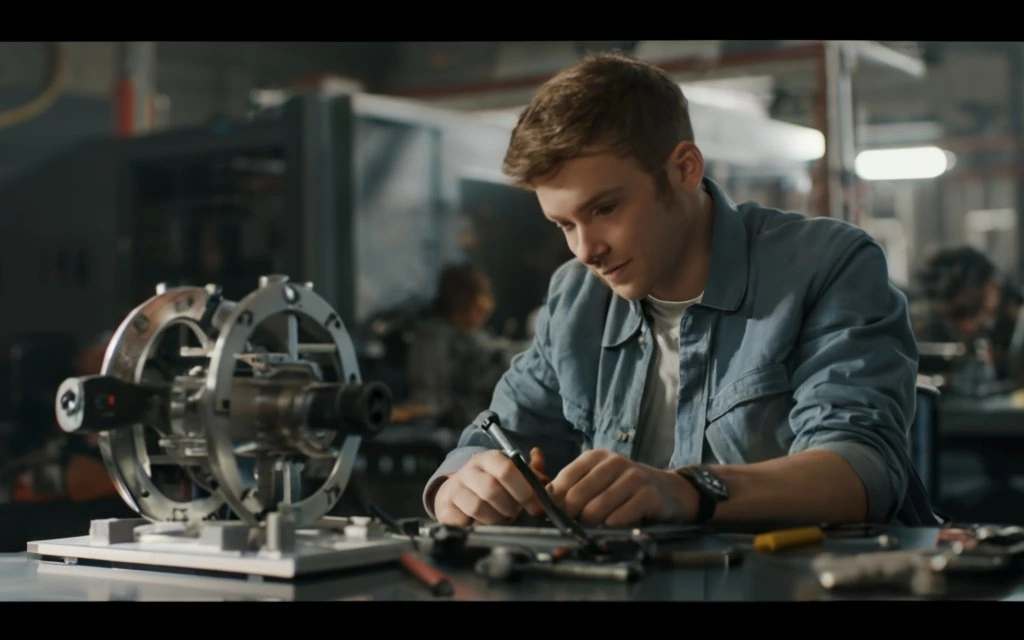
Another formidable challenge is the sheer breadth of mechanics as a subject. From statics and dynamics to fluid mechanics and thermodynamics, students must navigate a diverse landscape of concepts. This diversity can be overwhelming, making it imperative for students to discern the relevance of each sub-discipline to their specific assignments. Additionally, the time-consuming nature of mechanics assignments can exacerbate the challenges students face. As they grapple with intricate problems that require careful consideration and meticulous calculations, time management becomes a critical skill in ensuring both efficiency and accuracy.
Despite these challenges, the significance of overcoming them is paramount. Mechanics is not merely an academic exercise; it is the practical language spoken by mechanical engineers when designing, analyzing, and optimizing systems. Whether it be determining the structural integrity of a bridge, analyzing the motion of machinery, or designing a fluid flow system, a robust understanding of mechanics is the compass guiding engineers through the complexities of their projects.
In essence, this blog aims to provide practical tips that serve as a guiding light for students navigating the intricacies of mechanics assignments. By addressing the challenges head-on and offering insights into effective problem-solving strategies, students can cultivate a mastery of mechanics that extends beyond the theoretical realm. As we delve into the nuances of this subject, we embark on a journey to demystify mechanics, empowering students to not only meet the demands of their assignments but also to cultivate a profound appreciation for the indispensable role mechanics plays in shaping the world of mechanical engineering.
Understanding the Basics.
In the intricate world of mechanical engineering, a robust understanding of basic mechanical concepts serves as the linchpin for success. Akin to constructing a sturdy edifice, a strong foundation in mechanics is indispensable for students venturing into the complexities of this discipline. This foundation not only facilitates a seamless progression through advanced courses but also nurtures the analytical prowess required to solve real-world engineering problems. Like the roots of a mighty tree, the basics of mechanics anchor students in the principles that underpin the entire realm of mechanical engineering.
Newton's Laws of Motion.
Newton's Laws of Motion, the bedrock of classical mechanics, provide a comprehensive framework for understanding the dynamic behavior of objects in the physical world. Sir Isaac Newton's first law, often termed the law of inertia, elucidates that an object at rest will remain stationary, and an object in motion will persist in motion unless acted upon by an external force. The second law quantifies this relationship, stating that the force acting on an object is equal to the product of its mass and acceleration, thereby emphasizing the fundamental interplay between force, mass, and motion. Newton's third law introduces the principle of action and reaction, asserting that for every action, there is an equal and opposite reaction. This law underscores the symmetrical nature of forces in interactions, illustrating the interconnectedness of objects in the dynamic dance of the physical universe. Whether launching a rocket into space or understanding the subtleties of everyday motion, Newton's Laws provide a universal language, guiding engineers, physicists, and students alike through the intricacies of mechanical systems with enduring relevance and elegance.
Equilibrium in Systems.
Equilibrium in systems is a fundamental concept in mechanics, serving as the equilibrium state where the net forces and torques acting on an object or system are precisely balanced, resulting in a stable, unchanging condition. At the heart of this principle lies the delicate equilibrium equation: the sum of all forces must be zero for translational equilibrium, and the sum of all torques must be zero for rotational equilibrium. This equilibrium state ensures that an object remains at rest or moves at a constant velocity, providing a crucial foundation for analyzing structures and predicting the behavior of dynamic systems.
In practical terms, equilibrium allows engineers to assess the stability of structures, from bridges and buildings to intricate mechanical components. By meticulously considering the forces at play and ensuring their equilibrium, engineers can design structures that withstand external influences and maintain their integrity. Understanding equilibrium is akin to mastering the art of balance in the engineering realm, where precise calculations and strategic considerations come together to create structures that not only resist external forces but do so in a harmonious equilibrium state, embodying both stability and resilience.
Forces in Mechanical Systems.
Forces in mechanical systems are the unseen agents that drive motion, influence behavior, and maintain equilibrium in the dynamic world of engineering. These omnipresent forces play a pivotal role in shaping the trajectory and behavior of objects, from the grand scale of planetary motion to the intricacies of microscopic machinery. Understanding forces involves dissecting them into components, considering their direction and magnitude, and recognizing their effects on objects within a system.
Gravity, a ubiquitous force, governs the motion of celestial bodies and influences the weight of objects on Earth. Tension forces come into play when structures are under load, holding elements together and maintaining stability. Friction, a resistance force, opposes the relative motion of surfaces, impacting the efficiency of mechanical systems. Engineers meticulously analyze and manipulate these forces to optimize designs, ensuring structures remain in equilibrium and move with intended precision.
In the intricate dance of mechanical systems, forces serve as both challenges and tools, necessitating a profound comprehension for effective problem-solving and innovative design. From the propulsion of vehicles to the stability of structures, the nuanced understanding of forces empowers engineers to navigate the complexities of mechanical systems, transforming theoretical knowledge into practical solutions that propel the world of engineering forward.
Problem-Solving Approach.
Define the Problem and Identify Knowns and Unknowns:
Begin by clearly defining the problem at hand. Identify what is given (knowns) and what needs to be determined (unknowns). This step is crucial in establishing a roadmap for your solution and understanding the specific aspects of the mechanical problem you are addressing.
Choose an Appropriate Coordinate System:
Selecting a suitable coordinate system is essential for organizing and simplifying the problem. Whether Cartesian or polar, align the axes with the geometry of the system to streamline calculations. A well-chosen coordinate system minimizes complexities and enhances the clarity of your solution.
Apply Relevant Principles and Equations:
Draw upon the fundamental principles of mechanics that are relevant to the problem. This may involve Newton's laws, equilibrium equations, or specific principles related to the nature of the mechanical system. Translate the problem into a set of equations that express the relationships between knowns and unknowns.
Solve Step by Step, Showing All Calculations:
Systematically solve the equations derived from the application of principles. Perform each step of the solution process with precision, showing all intermediate calculations. This transparency not only aids in understanding but also allows for easy identification of errors, if any.
Check the Solution for Accuracy and Units:
Once the solution is obtained, perform a thorough check for accuracy. Verify that the solution aligns with the problem's requirements and expectations. Additionally, ensure that the units are consistent throughout the calculations, maintaining the integrity of the result.
This systematic problem-solving approach serves as a reliable guide for navigating the complexities of mechanical problems. By adhering to these steps, you not only enhance your understanding of the underlying principles but also cultivate a disciplined methodology that can be applied to a myriad of mechanical engineering challenges. Remember, meticulousness in each step is the key to deriving accurate and meaningful solutions.
Common Mistakes to Avoid.
In the realm of mechanical problem-solving, students often encounter pitfalls that, if unaddressed, can impede their understanding and hinder accurate solutions. One prevalent mistake is a lack of clarity in defining the problem and identifying known and unknown. Students may rush into calculations without a comprehensive understanding of the given information, leading to errors in the application of principles. It is imperative to meticulously define the problem parameters, ensuring a solid foundation for subsequent steps.
Another common misstep involves the choice of an inappropriate coordinate system. Selecting axes that do not align with the system's geometry can introduce complexities and convolute calculations. Precision in choosing a coordinate system streamlines the solution process and fosters a clearer representation of forces and motions within the system.
Misapplication of principles and equations is a recurrent challenge. Students may use incorrect formulas or misinterpret concepts, leading to faulty solutions. To mitigate this, a thorough understanding of the underlying mechanical principles is essential. Regular practice and familiarity with a range of problems contribute to a more nuanced application of relevant equations.
Neglecting units during calculations is a subtle yet impactful error. Inconsistent units can distort results and compromise the accuracy of the solution. A diligent review of units at each stage ensures that the final answer aligns with the problem's requirements and maintains dimensional consistency.
Inadequate step-by-step representation of calculations is a stumbling block. Skipping intermediate steps or failing to show the logic behind each calculation can hinder both self-review and the instructor's feedback. A clear and methodical presentation not only enhances understanding but also facilitates error detection and correction.
Lastly, overlooking the significance of units in the final answer is a critical error. Units are an integral part of the solution and provide context to the numerical result. Neglecting units can result in incomplete or misinterpreted solutions.
To mitigate these common mistakes, students should prioritize a systematic approach to problem-solving, emphasizing a comprehensive understanding of principles, precision in calculations, and meticulous attention to problem details. Regular practice, engagement with diverse problem sets, and seeking clarification on concepts contribute to a more adept and error-resilient approach to mechanical problem-solving. By addressing these common pitfalls, students can enhance their proficiency in tackling the intricacies of mechanical engineering problems.
Utilizing Resources.
In the dynamic realm of mechanics, effective problem-solving is not solely reliant on classroom instruction but is greatly enhanced by a strategic utilization of diverse resources. Encouraging students to harness the power of textbooks, online resources, and lecture notes can elevate their understanding and proficiency in tackling mechanical challenges.
Textbooks stand as foundational pillars in the learning process. They provide a structured and comprehensive exploration of mechanics principles, offering detailed explanations and a plethora of example problems. Emphasizing the importance of textbooks as essential references, students gain access to varied perspectives and additional insights. Diverse textbook exploration not only deepens understanding but also exposes students to different problem-solving approaches, broadening their analytical skills.
The digital landscape offers an array of resources that can dynamically augment traditional learning methods. Online platforms host interactive simulations, video tutorials, and supplementary materials that breathe life into theoretical concepts. Visualizations and virtual experiments found online bridge the gap between theory and application, offering students a practical understanding of abstract principles. Encouraging the exploration of reputable educational websites ensures that students have a well-rounded learning experience, tapping into the advantages of multimedia resources.
Lecture notes, crafted by instructors who tailor content to their teaching style, are invaluable resources. Encouraging students to actively engage with and organize their lecture notes transforms these materials into personalized study guides. Annotations, summarizations, and additional insights derived from lectures consolidate understanding and serve as a valuable reference during self-directed study sessions.
Undoubtedly, the importance of practice problems cannot be overstated. Engaging with a diverse range of problems hones problem-solving skills and reinforces theoretical knowledge. Regular practice not only builds confidence but also exposes students to the nuances of different problem types. It instills a resilient and adaptive approach to handling mechanical challenges, ensuring that students are well-equipped for the diverse problem landscapes they may encounter.
Encouraging students to proactively seek help when faced with challenges is a vital aspect of resource utilization. Whether through discussions with peers, consultations with instructors, or participation in study groups, seeking assistance fosters a collaborative and supportive learning environment. It transforms perceived weaknesses into opportunities for growth, ensuring that students' progress with a well-rounded understanding of mechanical concepts.
In conclusion, the effective utilization of textbooks, online resources, lecture notes, practice problems, and seeking help when needed forms a holistic approach to mastering mechanics. By integrating these resources strategically, students not only enhance their problem-solving skills but also cultivate a deeper and more enduring understanding of the principles that govern the fascinating world of mechanical engineering.
Conclusion:
In conclusion, the journey through the intricate landscape of mechanical engineering mechanics assignments necessitates not only a profound understanding of principles but also a strategic approach to problem-solving. The practical tips discussed in this blog—ranging from establishing a strong foundation in basics to utilizing diverse resources and embracing collaborative learning—aim to empower students in overcoming challenges systematically. By defining problems, choosing appropriate coordinate systems, and applying relevant principles, students lay the groundwork for successful solutions. The emphasis on leveraging resources such as textbooks, online materials, and lecture notes enriches their learning experience, providing varied perspectives and dynamic insights. Furthermore, the recognition of the importance of practice problems as a crucible for proficiency ensures that theoretical knowledge is honed into practical problem-solving skills.
Crucially, the encouragement to view seeking help as a strength fosters a collaborative and supportive learning environment, where challenges become opportunities for growth. As students embark on their mechanical engineering journey armed with these practical tips, they not only enhance their ability to navigate assignments effectively but also cultivate a mindset of adaptability and resilience—qualities indispensable in the ever-evolving field of engineering. In applying these practical tips, students are not merely tackling mechanics assignments; they are honing a skill set that extends beyond academia, preparing them for the challenges and innovations they will encounter in their future careers. May these insights serve as guiding principles, propelling students towards success and mastery in the captivating world of mechanical engineering.