Navigating Thermodynamics and Climate Challenges
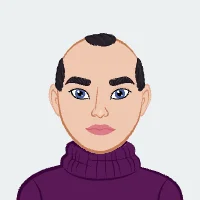
Thermodynamics and climate change assignments can be complex, but with a structured approach, you can master the concepts and effectively solve related problems. Here are some steps and strategies to help you tackle similar assignments:
Begin by thoroughly understanding the fundamental principles and theories that underlie thermodynamics, such as the laws of thermodynamics, the concepts of entropy, enthalpy, and Gibbs free energy, as well as the various thermodynamic cycles and processes. Next, familiarize yourself with the specific context of climate change and how thermodynamic principles apply to environmental systems and energy transfer mechanisms. Utilize diagrams and visual aids, such as P-V and T-S diagrams, to conceptualize and solve problems more effectively. Break down complex problems into manageable steps, and use systematic methods to analyze and solve each part of the assignment.
Regularly practice similar problems to reinforce your understanding and improve your problem-solving skills. Practice not only solidifies your grasp of key concepts but also helps you develop a systematic approach to solving problems, which is crucial for thermodynamics assignments. Additionally, leverage available resources such as textbooks, online courses, and professional thermodynamics assignment help services when needed to gain deeper insights and assistance. These resources can provide explanations, examples, and guidance that can clarify difficult topics and enhance your learning experience.
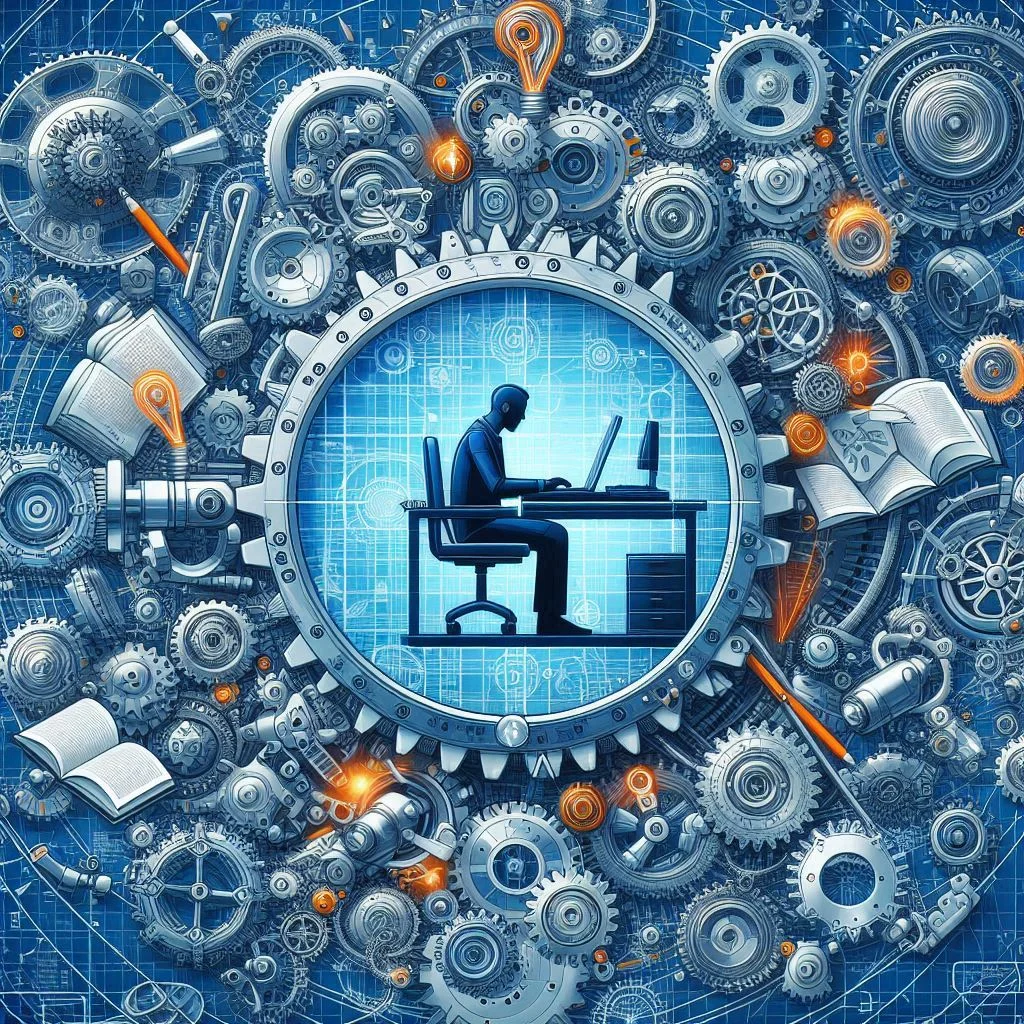
By following these strategies, you'll be well-prepared to handle a wide range of thermodynamics and climate change assignments. This structured approach will enhance both your knowledge and academic performance in this challenging yet fascinating field. Whether you are dealing with entropy changes, thermodynamic cycles, or the application of thermodynamics to climate change, a methodical and well-resourced approach will make your assignments more manageable and your understanding more profound. Utilizing thermodynamics assignment help when needed can provide the extra support necessary to excel in your studies.
Understanding Core Concepts
To effectively tackle assignments related to thermodynamics and climate change, it is essential to have a solid grasp of the core concepts underlying these topics. Understanding these fundamental principles will not only help you solve specific problems but also provide a framework for approaching a wide range of related issues. This section will delve into key concepts such as entropy and reversibility, thermodynamic cycles, and the use of P-V and T-S diagrams, all of which are crucial for mastering thermodynamics assignments. By building a strong foundation in these areas, you will be better prepared to analyze and solve complex problems, and to apply these principles to real-world scenarios involving energy transfer and environmental systems.
1. Entropy and Reversibility:
- Reversible Processes: These are ideal processes that happen infinitely slowly, maintaining equilibrium at every stage. In such processes, the entropy change of the system and surroundings is zero. For example, consider a gas expanding slowly within a cylinder fitted with a piston. If this expansion happens so slowly that the gas remains in thermal equilibrium with its surroundings at all times, the process is reversible. Understanding reversible processes is crucial because they represent the maximum efficiency limit that real processes can aim for.
- Irreversible Processes: These occur in real-world scenarios and involve factors like friction, unrestrained expansion, rapid mixing, and heat transfer with finite temperature differences, leading to a positive entropy change in the system and surroundings. For instance, when gas expands rapidly in a piston-cylinder assembly, it doesn’t have enough time to maintain equilibrium, resulting in irreversibilities such as turbulence and friction. Recognizing the sources of irreversibility helps in designing processes that minimize energy losses and improve efficiency.
2. Thermodynamic Cycles:
- Carnot Cycle: An idealized thermodynamic cycle proposed by Sadi Carnot, which provides the maximum possible efficiency for a heat engine operating between two temperature reservoirs. The Carnot cycle involves two isothermal processes (heat transfer at constant temperature) and two adiabatic processes (no heat transfer). By understanding the Carnot cycle, you can analyze and compare the efficiency of real engines and refrigeration systems.
- Real Cycles: Practical thermodynamic cycles such as the Rankine cycle (used in power plants), the Otto cycle (used in internal combustion engines), and the refrigeration cycle (used in refrigerators and air conditioners) are deviations from the ideal Carnot cycle due to practical constraints. Learning about these cycles helps in understanding the functioning, efficiency, and optimization of real thermal systems.
3. P-V and T-S Diagrams:
- P-V Diagram: A plot of pressure (P) versus volume (V) that is useful for visualizing processes such as isochoric (constant volume), isobaric (constant pressure), isothermal (constant temperature), and adiabatic (no heat transfer). For example, in an isothermal expansion, the gas expands at a constant temperature, and the P-V curve will be a hyperbola. Understanding P-V diagrams aids in the analysis of work done by or on the system during different thermodynamic processes.
- T-S Diagram: A plot of temperature (T) versus entropy (S) that helps in visualizing heat transfer processes and the efficiency of cycles. For instance, the area under a process curve in a T-S diagram represents the heat transferred during that process. By analyzing T-S diagrams, you can better understand the thermal efficiency of cycles and the entropy changes involved.
By mastering these core concepts and learning to apply them to various thermodynamic systems and processes, you'll be better equipped to tackle assignments and problems related to thermodynamics and climate change. This foundational knowledge will enable you to approach complex scenarios systematically and find effective solutions.
Solving Problems Step-by-Step
Approaching thermodynamics and climate change assignments systematically can make complex problems more manageable. Here’s a step-by-step guide to help you solve such assignments effectively:
1. Analyzing Entropy Changes:
- For Reversible Processes: Use the second law of thermodynamics and the definition of entropy change (ΔS=∫dQrevT\Delta S = \int \frac{dQ_{rev}}{T}ΔS=∫TdQrev) to determine the change in entropy. For instance, in an isothermal process, where the temperature remains constant, you can simplify the calculation by integrating over the constant temperature.
- For Irreversible Processes: Consider additional factors like friction, rapid expansion, and mixing, which contribute to entropy production. Use the principle that the entropy change of the universe (system + surroundings) is always positive for irreversible processes.
2. Applying Thermodynamic Relations:
- Use specific heat capacities at constant volume (CvC_vCv) and constant pressure (CpC_pCp) to relate changes in entropy, temperature, volume, and pressure. For example, for a perfect gas, the change in entropy can be expressed as: ΔS=Cvln(T2T1)+Rln(V2V1)\Delta S = C_v \ln \left( \frac{T_2}{T_1} \right) + R \ln \left( \frac{V_2}{V_1} \right)ΔS=Cvln(T1T2)+Rln(V1V2) and similarly, ΔS=Cpln(T2T1)−Rln(P2P1)\Delta S = C_p \ln \left( \frac{T_2}{T_1} \right) - R \ln \left( \frac{P_2}{P_1} \right)ΔS=Cpln(T1T2)−Rln(P1P2)
- These equations help relate state variables and make it easier to solve for unknowns in a given problem.
3. Working with Thermodynamic Cycles:
- Carnot Cycle: Sketch the cycle on a P-V or T-S diagram. For each segment (isothermal expansion, adiabatic compression, etc.), calculate the work done and heat transferred. For example, during an isothermal expansion, use W=nRTln(VfVi)W = nRT \ln \left( \frac{V_f}{V_i} \right)W=nRTln(ViVf) to find the work done by the gas.
- Real Cycles: Understand the deviations from the ideal cycles due to irreversibilities and practical constraints. Calculate the efficiency of real cycles and compare it with the Carnot efficiency to identify areas for improvement.
4. Utilizing Diagrams:
- P-V Diagrams: These help in visualizing processes such as isochoric, isobaric, isothermal, and adiabatic processes. Plotting these on a P-V diagram can provide insights into the nature of the processes and the work interactions involved.
- T-S Diagrams: Use these to visualize heat transfer processes and understand the changes in entropy. The area under a process curve on a T-S diagram represents the heat transferred, which can help in analyzing the thermal efficiency of cycles.
5. Practical Application and Design:
- Designing a Solar-Powered Heat Pump: Use the Carnot cycle as a reference to design efficient cooling systems. Calculate the necessary work input and the resulting Coefficient of Performance (COP) based on the temperature difference between the inside of the house and the environment. Sketch the cycle on a P-V diagram, label states, and calculate work and heat transfer for each process.
6. Coding and Simulations:
- Using Tools and Libraries: Use computational tools and libraries, such as the NASA Polynomial library, to model real gases and calculate entropy changes for various processes. For instance, set up a code to iteratively solve for equilibrium states and entropy changes in gas mixing problems.
- Simulation Projects: Engage in projects that require coding to simulate thermodynamic processes. This not only enhances your understanding but also prepares you for practical applications in research and industry.
By following these step-by-step strategies, you can systematically approach and solve thermodynamics and climate change assignments. This methodical approach ensures that you cover all aspects of the problem, leading to more accurate and comprehensive solutions.
Practical Application
Applying theoretical knowledge to real-world scenarios is a crucial aspect of mastering thermodynamics and climate change assignments. Here are some practical applications to help you bridge the gap between theory and practice:
1. Designing Energy-Efficient Systems:
- Solar-Powered Heat Pumps: As the Earth warms, designing efficient cooling systems becomes increasingly important to mitigate climate change effects. A solar-powered heat pump can be designed using the principles of the Carnot cycle, which is an idealized thermodynamic cycle that provides the maximum possible efficiency.
Example: Consider a house as a thermal reservoir at TL=20∘CT_L = 20^\circ CTL=20∘C and the outside environment at TH=35∘CT_H = 35^\circ CTH=35∘C. The heat pump uses solar energy to power an isentropic compressor. Sketch the cycle on a P-V diagram, label states 1-4, and indicate the processes (isothermal expansion, isentropic compression, etc.). Calculate the work and heat transfer for each process. Determine the mass flow rate needed to maintain a constant temperature if 500 W of heat is added to the house consistently. Discuss how to minimize the amount of working fluid by selecting a fluid with appropriate heat capacity.
2. Analyzing Real-World Processes:
- Refrigeration and Air Conditioning: Understanding the thermodynamic cycles involved in refrigeration and air conditioning systems can help optimize their performance and reduce energy consumption.
Example: Analyze a typical refrigeration cycle, including the processes of compression, condensation, expansion, and evaporation. Use T-S diagrams to visualize the cycle and calculate the COP. Identify sources of irreversibility, such as friction and non-ideal gas behavior, and suggest methods to reduce entropy generation and improve system efficiency.
3. Environmental Impact Assessment:
- Greenhouse Gas Emissions: Thermodynamics principles can be applied to assess the impact of different energy systems on greenhouse gas emissions.
Example: Evaluate the carbon footprint of various power generation methods, such as coal, natural gas, and renewable energy sources. Calculate the efficiency of each method using thermodynamic principles and compare the amount of CO2 emitted per unit of energy produced. Discuss how improvements in thermodynamic efficiency can lead to significant reductions in emissions.
4. Sustainable Energy Solutions:
- Renewable Energy Integration: Applying thermodynamics to the integration of renewable energy sources, such as solar and wind, into existing energy systems can enhance sustainability.
Example: Design a system that combines solar panels with a heat storage unit. Use thermodynamic principles to calculate the efficiency of the system and the amount of energy stored. Analyze the heat transfer processes involved and optimize the design to maximize energy storage and minimize losses.
5. Coding and Simulation:
- Entropy Calculation Using Software: Utilize computational tools to simulate thermodynamic processes and calculate entropy changes.
Example: Using a library like NASA's Polynomial library, write a code to calculate the change in entropy for a gas undergoing different processes. For instance, model the expansion of carbon dioxide from 600 K to 400 K with a pressure drop from 10 bar to 1 bar. Compare the results from the simulation with theoretical calculations to validate the model.
6. Climate Change Mitigation Technologies:
- Carbon Capture and Storage (CCS): Thermodynamics plays a crucial role in designing efficient CCS technologies to reduce atmospheric CO2 levels.
Example: Design a thermodynamic cycle for a carbon capture system that uses a solvent to absorb CO2 from flue gas. Analyze the energy requirements for the absorption and regeneration processes. Calculate the efficiency of the system and propose methods to reduce energy consumption and increase CO2 capture efficiency.
By applying these practical examples, you can see how thermodynamic principles are used to solve real-world problems, improve system efficiencies, and develop sustainable technologies. This not only enhances your understanding of theoretical concepts but also prepares you to address pressing global challenges related to energy and climate change.
Additional Tips
To further enhance your ability to handle complex thermodynamics and climate change assignments, consider these additional tips:
1. Stay Organized:
- Outline Your Work: Before diving into solving the assignment, outline the steps you need to take. This helps in breaking down the problem into manageable parts.
- Keep a Formula Sheet: Maintain a sheet with key formulas and constants. This quick reference can save time and ensure accuracy.
2. Master the Basics:
- Fundamental Equations: Ensure you have a strong grasp of fundamental equations such as the first and second laws of thermodynamics, the ideal gas law, and relations for entropy, enthalpy, and internal energy.
- Unit Consistency: Always check the units of your calculations to avoid errors. Convert units where necessary to maintain consistency throughout your solution.
3. Use Resources Wisely:
Textbooks and Online Courses: Utilize textbooks and online platforms like MIT OpenCourseWare and Coursera for in-depth explanations and examples.
1. Thermodynamics Assignment Help: Don’t hesitate to seek professional help if you’re stuck. Services specializing in thermodynamics can provide guidance and detailed solutions.
2. Practice Regularly:
- Work on Example Problems: Regular practice with a variety of problems enhances your understanding and problem-solving skills. Focus on problems that challenge different aspects of thermodynamics.
- Simulate Real-World Scenarios: Try to simulate real-world problems using software tools like MATLAB, Python, or specialized thermodynamics software. This practical experience can be invaluable.
3. Collaborate and Discuss:
- Study Groups: Join or form study groups to discuss concepts and solve problems together. Explaining concepts to peers can deepen your understanding.
- Online Forums: Participate in online forums and communities like Stack Exchange or Reddit. These platforms allow you to ask questions and share knowledge with others.
4. Visualize Problems:
- Draw Diagrams: Always sketch diagrams for processes and cycles. Visual aids like P-V and T-S diagrams help in understanding the relationships between different thermodynamic quantities.
- Use Graphing Tools: Leverage graphing tools to plot functions and visualize data. This can make it easier to identify trends and interpret results.
5. Develop a Systematic Approach:
- Identify Knowns and Unknowns: Clearly list out the given data and the variables you need to find. This clarity helps in formulating a plan to solve the problem.
- Check Your Work: After solving the problem, review each step to ensure there are no mistakes. Verify your results by cross-checking with different methods if possible.
6. Stay Updated:
- Latest Research: Keep abreast of the latest research and developments in thermodynamics and climate science. Journals and conferences can provide insights into new techniques and applications.
- Emerging Technologies: Learn about emerging technologies in energy systems and climate mitigation. Understanding how thermodynamics principles are applied in innovative solutions can broaden your perspective.
By incorporating these additional tips into your study routine, you can enhance your problem-solving skills, improve your understanding of thermodynamics, and become more adept at handling assignments related to climate change. This comprehensive approach will not only help you excel academically but also prepare you for real-world applications and challenges in the field of thermodynamics.
Conclusion
In conclusion, mastering thermodynamics and its applications to climate change is both a challenge and a rewarding endeavor. By understanding the fundamental principles, such as the laws of thermodynamics, entropy, and thermodynamic cycles, you gain the tools to analyze and solve complex problems effectively.
Throughout this guide, we've explored systematic approaches to tackling thermodynamics assignments, emphasizing the importance of:
- Understanding Core Concepts: From entropy changes to thermodynamic cycles, a strong foundation is essential.
- Practical Application: Applying theory to real-world scenarios like designing solar-powered heat pumps and assessing environmental impacts.
- Additional Tips: Organizing your work, practicing regularly, using resources wisely, and visualizing problems.
By following these strategies and tips, you can navigate through challenging thermodynamics assignments with confidence. Remember, continuous practice, collaboration, and staying updated with advancements in the field are key to mastering thermodynamics and contributing to solutions for global challenges like climate change.