Understanding Entropy and Energy: In-Depth Analysis for Thermodynamics Assignments!
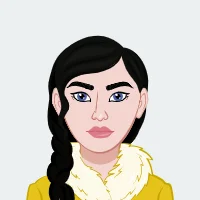
Thermodynamics stands as the cornerstone of mechanical engineering, providing the fundamental framework for understanding the behavior of energy and matter within systems. In the dynamic realm of mechanical engineering, thermodynamics serves as an essential tool, governing the principles that drive the design, analysis, and optimization of various mechanical systems. At its core, thermodynamics delves into the principles of energy transfer and transformation, shedding light on the intricate relationships between heat, work, and the properties of matter. If you need help with your thermodynamics assignment, mastering the principles of thermodynamics is crucial for solving complex engineering problems and designing efficient mechanical systems, making it a foundational aspect of mechanical engineering education.
Within the expansive domain of thermodynamics, entropy and energy emerge as pivotal concepts, shaping the way engineers comprehend and manipulate systems. Energy, a fundamental property of matter, manifests in various forms, including kinetic, potential, and internal energy. Thermodynamics provides engineers with the framework to quantify and analyze energy changes within a system, allowing for the efficient design and optimization of mechanical devices. Entropy, on the other hand, introduces the concept of disorder within a system. As entropy increases, the degree of disorder rises, influencing the efficiency and feasibility of energy transformations. Understanding and manipulating entropy is essential for engineers seeking to enhance the performance and efficiency of mechanical systems.
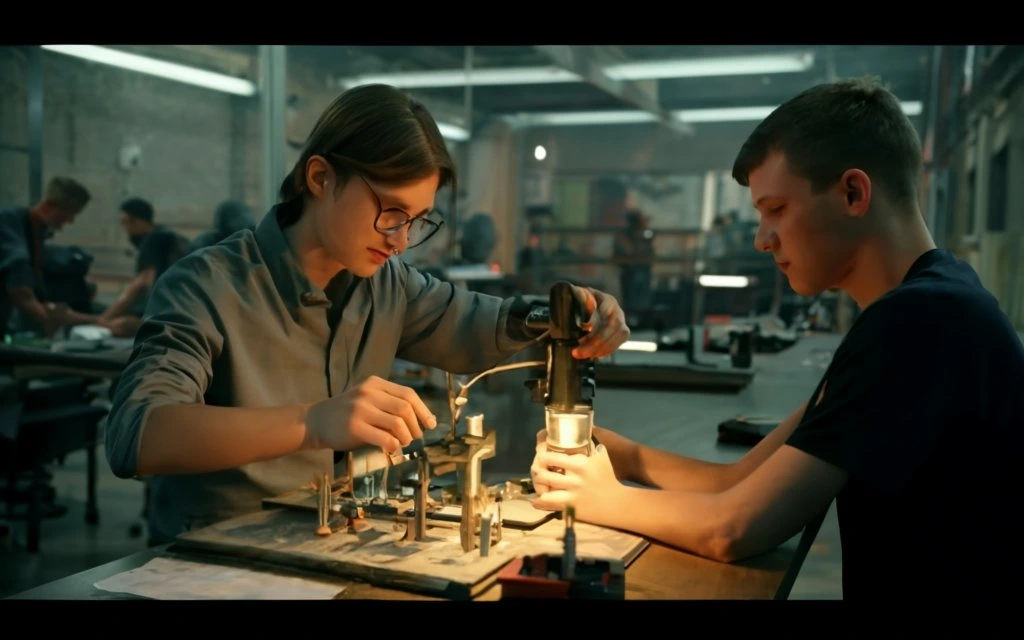
A profound grasp of these thermodynamic concepts is indispensable for students navigating the challenges of mechanical engineering assignments. The ability to comprehend and apply the laws of thermodynamics, such as the conservation of energy and the increase of entropy, is paramount for success in solving complex engineering problems. Assignments often present scenarios where a meticulous understanding of energy flow and entropy changes is required to devise innovative solutions. Without a solid foundation in thermodynamics, students may find themselves grappling with intricate assignments that demand a nuanced comprehension of energy principles.
In the realm of mechanical engineering assignments, the significance of entropy and energy becomes particularly pronounced. Tasks involving heat exchangers, engines, refrigeration systems, and power plants necessitate a meticulous application of thermodynamic principles. Students are often tasked with analyzing and optimizing these systems, requiring a deep understanding of how energy is transferred and transformed. Furthermore, assignments may involve addressing challenges related to increasing entropy, balancing energy equations, or designing systems with maximal efficiency. A solid grasp of thermodynamics equips students with the analytical tools needed to navigate such assignments successfully.
In conclusion, thermodynamics stands as an integral discipline within the field of mechanical engineering, providing the foundational principles for understanding energy and matter in systems. Entropy and energy, as key concepts within thermodynamics, shape the framework for comprehending the behavior of mechanical systems. A profound understanding of these concepts is not only vital for engineers designing and optimizing mechanical systems but is also crucial for students striving to excel in their assignments. The ability to apply thermodynamic principles with precision empowers students to unravel the complexities of mechanical engineering challenges, fostering a deeper appreciation for the interconnected world of energy and matter.
Basics of Thermodynamics
Thermodynamics, a cornerstone in the realm of physics and engineering, is a branch that delves into the principles governing energy, heat, and their interactions within physical systems. At its core, thermodynamics provides a framework for understanding and predicting the behavior of matter and energy, making it an indispensable discipline in the field of mechanical engineering. Central to thermodynamics is the concept of a system, which refers to a defined portion of the universe under scrutiny, and its surroundings, constituting the rest of the universe outside the system's boundaries. The interactions between the system and its surroundings, involving the exchange of energy and matter, form the basis of thermodynamic analysis.
Key terms in thermodynamics include energy, a fundamental property that exists in various forms such as kinetic, potential, and internal energy. The laws of thermodynamics, a set of fundamental principles governing energy transformations, are paramount in this discipline. The First Law of Thermodynamics, often referred to as the law of energy conservation, asserts that energy cannot be created or destroyed; it can only change forms. This foundational law has profound implications for mechanical engineering, guiding the understanding of energy transfer and transformation within machines and systems.
The Second Law of Thermodynamics introduces the concept of entropy, a measure of the degree of disorder or randomness in a system. This law emphasizes the natural tendency of systems to move towards a state of increased entropy, signifying the irreversibility of certain processes. In the context of mechanical engineering, this law is crucial for assessing the efficiency and performance of machines, as it highlights the limitations imposed by inherent thermodynamic constraints.
Thermodynamics is fundamental to mechanical engineering for several reasons. Firstly, it provides the theoretical underpinning for the design and analysis of engines, heat exchangers, and other mechanical systems. Engineers rely on thermodynamic principles to optimize the efficiency of these systems, ensuring they operate in a manner consistent with the laws governing energy transformations. Additionally, thermodynamics plays a pivotal role in the understanding of heat transfer mechanisms, allowing engineers to design effective cooling and heating systems essential in various applications.
In conclusion, thermodynamics serves as the bedrock of mechanical engineering, offering a comprehensive framework for analyzing energy interactions within systems. The systematic application of the laws of thermodynamics facilitates the design, optimization, and analysis of mechanical systems, making it an indispensable discipline in the pursuit of engineering excellence.
Introduction to Entropy and Energy
In the realm of thermodynamics, entropy and energy are pivotal concepts that underpin the behavior of systems and processes. Entropy, often referred to as the measure of disorder or randomness in a system, plays a crucial role in understanding the spontaneous nature of physical processes. In simpler terms, as entropy increases, so does the level of disorder within a system. This concept is intricately connected to the Second Law of Thermodynamics, which states that natural processes tend to move towards states of higher entropy, emphasizing the inherent tendency of systems to evolve into more disordered configurations over time.
Energy, on the other hand, is the fundamental currency of the universe and a central theme in thermodynamics. It exists in various forms, and its transformations are governed by the laws of thermodynamics. In mechanical systems, energy is classified into two main types: kinetic and potential. Kinetic energy is associated with the motion of objects, while potential energy is linked to their position or state. These energy forms are interconnected, and the conversion between them is exemplified by the First Law of Thermodynamics, stating that energy in a closed system is conserved.
The relationship between entropy and disorder becomes evident when considering energy transformations. As energy undergoes conversions within a system, there is often a tendency for it to disperse and distribute, leading to an increase in entropy. For instance, in a combustion process, the chemical potential energy stored in fuel molecules is converted into thermal energy, which then disperses, increasing the overall entropy of the system.
Mechanical systems, being a subset of thermodynamic systems, heavily rely on the interplay of various forms of energy. In addition to kinetic and potential energy, mechanical systems frequently involve heat and work interactions. Heat is the transfer of thermal energy due to a temperature difference, while work is the energy transferred by a force acting through a distance. Understanding these energy forms is crucial in analyzing and designing mechanical systems, as it allows engineers to optimize performance and efficiency.
In conclusion, entropy and energy are cornerstone concepts in thermodynamics, offering profound insights into the behavior of systems. The relationship between entropy and disorder, coupled with the diverse forms of energy in mechanical systems, provides a robust framework for comprehending the intricacies of energy transformations. This foundational knowledge not only deepens our understanding of the natural world but also serves as a guiding principle for engineers in their quest to harness and manipulate energy for practical applications.
The First Law of Thermodynamics
The First Law of Thermodynamics stands as a foundational principle in the realm of thermodynamics, encapsulating the concept of energy conservation within closed systems. At its core, this law asserts that energy cannot be created nor destroyed; instead, it undergoes various transformations within a system. In the context of mechanical systems, the First Law serves as a guiding principle, dictating the behavior and interactions of energy.
The conservation of energy, as stipulated by the First Law, implies that the total energy within an isolated system remains constant over time. This conservation principle holds true for mechanical systems, emphasizing that the sum of kinetic, potential, and internal energy remains unaltered unless acted upon by external forces or sources. In essence, energy can change forms—shifting from kinetic to potential, for instance—yet the overall quantity persists, showcasing the inherent stability of energy in mechanical processes.
To elucidate the application of the First Law in mechanical systems, consider the classic example of a piston-cylinder arrangement. As a piston compresses a gas within a cylinder, the system undergoes changes in pressure, volume, and temperature. According to the First Law, the increase in internal energy of the gas is equal to the work done on it and the heat added to the system. This relationship is encapsulated in the equation ΔU = Q - W, where ΔU is the change in internal energy, Q represents the heat added to the system, and W signifies the work done on the system.
Furthermore, envision a scenario where a rotating flywheel imparts its kinetic energy to a connected mechanical system. As the flywheel transfers its energy, the mechanical components, driven by the laws of the First Law, undergo transformations without a net loss or gain in total energy. This adherence to energy conservation is not only pivotal in theoretical considerations but also finds practical applications in designing efficient mechanical systems, such as engines and power generation plants.
In conclusion, the First Law of Thermodynamics, epitomizing the conservation of energy, serves as a cornerstone in understanding the behavior of energy in mechanical systems. Its application is instrumental in unraveling the intricacies of processes like compression in pistons or energy transfer in rotating systems. By embracing the principles of energy conservation, mechanical engineers gain invaluable insights into the dynamics of systems and can adeptly design solutions that optimize energy utilization within the constraints dictated by the First Law.
The Second Law of Thermodynamics
Entropy is a crucial concept in thermodynamics, playing a pivotal role in the Second Law, which governs the direction of natural processes. Entropy is a measure of the disorder or randomness in a system. In the context of the Second Law, it states that the total entropy of an isolated system tends to increase over time. This increase in entropy implies a movement towards a state of greater disorder or randomness.
The role of entropy in the Second Law is closely tied to the idea of irreversibility. Natural processes tend to move towards states with higher entropy, showcasing the inherent tendency of systems to evolve towards chaos. This is in stark contrast to the idealized scenarios in the First Law of Thermodynamics, where energy is conserved and processes are reversible. The Second Law introduces a certain directionality to processes, emphasizing the arrow of time and the inevitability of systems proceeding towards states of greater disorder.
Implications of the Second Law for energy transfer and transformation are profound. It implies that not all the energy in a system can be converted into useful work. Some energy will always be lost in the form of heat, leading to an increase in entropy. This limitation has significant consequences for the efficiency of various energy conversion processes. For instance, in a heat engine, the Second Law dictates that not all the heat energy extracted from a hot reservoir can be converted into mechanical work. Some of it must be rejected as waste heat to a colder reservoir, increasing the overall entropy of the system.
Real-world examples vividly illustrate the Second Law's impact on energy processes. Consider the combustion of fossil fuels in an internal combustion engine. The chemical energy stored in the fuel is released as heat, but not all of it can be transformed into useful work to propel the vehicle. A significant portion is inevitably lost as exhaust heat, contributing to the overall increase in entropy. Similarly, electricity generation through power plants faces the challenge of heat dissipation, adhering to the Second Law as it imposes limits on the efficiency of converting heat into electrical energy.
In conclusion, understanding entropy and its role in the Second Law is fundamental to comprehending the behavior of natural processes. The law's implications for energy transfer and transformation underscore the challenges inherent in harnessing energy efficiently. Real-world examples serve as tangible illustrations, emphasizing the practical significance of these theoretical principles in the field of mechanical engineering.
Applications in Mechanical Engineering Assignments
A profound comprehension of entropy and energy is pivotal for mastering thermodynamics assignments, as these concepts form the bedrock of this branch of physics and have far-reaching implications in the realm of mechanical engineering. Entropy, often described as a measure of disorder in a system, and energy, in its various forms, play integral roles in the laws governing energy transfer and transformation within mechanical systems. For students delving into thermodynamics assignments, a solid grasp of these principles unlocks the door to solving complex problems and designing innovative solutions.
Typical thermodynamics assignments often revolve around the application of the First and Second Laws. One common task involves analyzing heat and work interactions within a given system, requiring students to apply the First Law to account for energy conservation. Another frequent assignment entails assessing the efficiency of thermodynamic processes, wherein students must navigate the intricacies of entropy changes to determine the viability of energy transformations. Real-world applications might involve the design of heat exchangers, power cycles, or refrigeration systems, demanding a comprehensive understanding of entropy and energy principles for successful completion.
Approaching thermodynamics assignments effectively necessitates a systematic strategy. Firstly, students should strive to comprehend the theoretical foundations thoroughly, delving into the mathematical formulations and physical interpretations of entropy and energy. Visualization tools, such as diagrams and charts, can aid in conceptualizing abstract ideas. Secondly, practical application is key. Linking theoretical knowledge to real-world scenarios fosters a deeper understanding and enhances problem-solving capabilities. Thirdly, breaking down complex problems into smaller, manageable components facilitates a step-by-step approach, enabling students to tackle intricate thermodynamics assignments with confidence.
Moreover, collaboration and seeking assistance when needed are invaluable strategies. Engaging in discussions with peers, consulting professors, or availing oneself of online resources can provide fresh perspectives and insights. Embracing a proactive attitude towards learning, including hands-on experiments or simulations, reinforces theoretical concepts. Time management is crucial, allowing students to allocate sufficient time to grasp challenging aspects of entropy and energy, ensuring a thorough understanding before attempting problem-solving.
In conclusion, the mastery of entropy and energy concepts is not only a prerequisite for success in thermodynamics assignments but is foundational for aspiring mechanical engineers. Real-world applications abound, demanding a nuanced understanding of these principles. By embracing a systematic approach, applying theoretical knowledge to practical scenarios, and seeking collaborative learning opportunities, students can navigate the complexities of thermodynamics assignments with proficiency, paving the way for a deeper appreciation of mechanical engineering principles and practices.
Conclusion:
In this comprehensive blog post titled "Understanding Entropy and Energy: In-Depth Analysis for Thermodynamics Assignments," we delved into the fundamental concepts of thermodynamics and their crucial role in mechanical engineering. Beginning with an overview of thermodynamics, we explored the significance of entropy and energy, highlighting their foundational importance in the field. Entropy, a measure of disorder, and the various forms of energy were elucidated, setting the stage for a deeper analysis.
The discussion progressed to a thorough examination of the First and Second Laws of Thermodynamics. The First Law, addressing energy conservation, was scrutinized for its implications in mechanical systems, while the Second Law, with its focus on entropy, shed light on the inherent nature of energy transfer and transformation. Real-world examples were incorporated to illustrate these complex concepts, providing readers with a practical understanding.
Importantly, the blog post emphasized the practical applications of entropy and energy in mechanical engineering assignments. A profound understanding of these concepts is portrayed as essential for tackling thermodynamics assignments effectively. By providing insight into the intricacies of the laws and their applications, students are better equipped to navigate and solve challenging problems related to entropy and energy in their coursework.
In conclusion, the key takeaway is the paramount importance of a deep understanding of entropy and energy in the realm of mechanical engineering. Mastery of these concepts not only enriches theoretical knowledge but also equips students with the analytical tools needed to excel in their assignments and future endeavors in the field. Recognizing the challenges that students may face, we extend an invitation for readers to explore our website, mechanicalengineeringassignmenthelp.com, for additional resources and expert assistance with their assignments. Our platform aims to be a valuable support system, offering comprehensive guidance and specialized services tailored to the unique demands of mechanical engineering coursework. As students embark on their academic journeys, we stand ready to empower them with the knowledge and tools necessary to thrive in the dynamic and rewarding field of mechanical engineering.